The Power of Food
Engineering Connection
Electrical engineers apply their knowledge of electrical circuits to design solutions (such as creating lights) to defined problems (such as alerting a rescue airplane). Engineers must completely understand the difference between series and parallel circuits as well as the behavior of many other components that can be put into circuits, such as resistors, diodes and capacitors. An example of this is a cell phone, for which an electrical engineer must create a tiny circuit on a computer chip. In the chip are many components that manipulate the current in many ways to cause the phone to do certain things when the buttons are pushed. The same is true for a touch screen phone except sensors are used to detect the location you are touching. Electrical engineers also manipulate the voltage coming out of a wall (electrical) outlet so that the correct amount of voltage is released without burning up (or overheating) whatever is plugged into the socket—such as cell phone chargers. This is accomplished with a circuit and electrical components organized inside of the charger. Often, when designing new products, such as in this activity, engineers are limited by the materials available.
Basic Knowledge
- The parts of a series circuit are connected in succession, and total voltage of the circuit is equal to the sum of the voltage across each part of the circuit. A parallel circuit has its parts connected with two wires entering and two wires exiting each part. In a parallel circuit, the same voltage is applied to each part of the circuit.
- In a series circuit, current does not have to split among components, and thus, the current through each component in the circuit is equal. In a parallel circuit, current splits at each new component and then returns at the end of each split. The total current in a parallel circuit is equal to the sum of the current through each component of the circuit. In parallel circuit, the voltage splits among the "ladder" of resistors.
- If the lights are in series, the current across each LED will be equal to the circuit's total current.
- When the LEDs are in parallel, the current is split among the pathways in the circuit, thus each light has a smaller current passing through it in a parallel circuit as opposed to a series circuit.
Figure 1. Schematic illustration of a parallel circuit (left) and a series circuit (right).
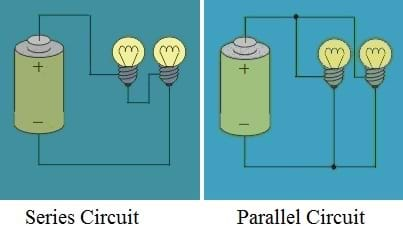
Series Circuit
A series circuit is one with all the loads in a row. There is only ONE path for the electricity to flow. If this circuit was a string of light bulbs, and one blew out, the remaining bulbs would turn off.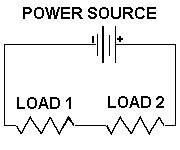
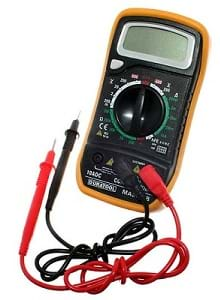
Vocabulary/Definitions
amperes: Measurement of the amount of electrical current passing a point over a given times. Current is measured in amperes (amps).
current: The flow of electrons in a system. The system can be anything that acts as a conductor.
diode: A device that contains a negative (anode) and a positive (cathode) component. Because of the positive and negative sides, a diode only conducts current in one direction. An LED is an example of a diode that is used in this activity.
LED: An LED is a diode; therefore current can only pass through it in one direction. As the current passes through an LED in the correct direction (positive to negative), it emits visible light.
parallel circuit: A closed electrical circuit in which the current is divided into two or more paths and then returns via a common path to complete the circuit.
series circuit: A circuit having its parts connected in successive parts.
voltage: The difference in electrical potential between two points; a measurement of 10 volts means that the two points measured have a difference of electrical potential equal to 10 volts.
Procedure
Background
Before the Activity
- Decide how to divide the class into groups composed of students with varying skill levels.
- Gather the materials, and portion them equally for each group.
With the A Team
- Tell teams that their challenge is to create a battery that is as powerful as possible from fruit, potatoes and assorted liquids. Give A Team two of each type of food and 5 cups to test different liquids.
- Pass out the worksheet, and give A Team time and assistance in completing the first page.
- Divide the class into groups and have them read the pre-activity reading. Direct students to refer to the reading when setting up their circuits. They must use the metal materials (pennies, nickels, paper clips and galvanized nails) to place inside the fruit, potato, soda or bleach, and then connect these metal materials in the circuit using wire and alligator clips. Have one student in each group, wearing safety glasses, handle putting the metal in the liquid items.
- Have A Team brainstorm ideas and write an initial strategy for their circuits. Check in with each group during this time period to ensure they are on task.
- Once each group has put together a plan, give teams adequate time to use the provided materials to build and test their circuits.
- As they try different things, direct A Team to take careful notes in their engineering journals of their findings, so they can remember what worked well and what did not.
- Circulate the room to ensure students are building, or attempting to build, viable circuits. (Note: series circuits work better than parallel circuits.) Also, be sure that the students are correctly placing the orientation of negative and positive ends of the fruits (see Figure 4). If students are having difficulty with their circuits, refer to the Troubleshooting Tips section for suggestions.
Figure 4. In a lemon battery cell, the copper wire is the positive electrode and the steel wire is the negative electrode. - After about 30 minutes, have A Team come together as a class and share their ideas. Have each group explain its initial strategy and the maximum voltage reached and the total number of lights lit. (Note: In tests, groups generated 1-12 volts, with 1-7 LEDs lit. It may be necessary to lower the lights in the room to see the LEDs lit at a dim level. Be aware that one LED lit brightly is equivalent to many lit very dimly; however, do not tell A Team this; let them get very excited about lighting more lights even at dim levels. The fact that they are still generating the same voltage as a brightly lit light is a good post-activity discussion topic.)
- Give A Team a new challenge: create the most voltage possible from one single food. (Note: In tests, students were able to generate 1-9 volts and could light 1-5 LEDs.)
- After about 30 minutes, have A Team come together as a class. Have each group explain its strategy, the maximum voltage reached and the number of lights lit.
- Ask the remainder of the questions provided in the Post-Activity Assessment section. If time permits, ask the Investigating Questions.
Safety Issues
- Handle bleach, fruit juice and vinegar with care, so that the liquids do not get in anyone's eyes. Make sure that just one student per group puts metal objects into the liquid, and enforce the wearing of safety glasses by these A Team .
- The potential danger of shock exists during the activity, although the shock would be minimal due to the low voltage of the circuits.
Troubleshooting Tips
If A Team get a negative reading for voltage at any point in the activity, it means they have the leads backwards and simply need to switch them.Common mistakes to be looking for if circuits are not working:
- Must be positive to negative to positive, to negative, etc. As shown in Figure 4 the negative end is the nail or paperclip, and the positive end is the penny or copper wire.
- The orientation of the LED (diode) can be confusing because it has both a negative and positive end. The positive end is the longer end of the diode wires. The positive and negative ends must be orientated the same way as the positive and negative terminals of every other component.
- The amount of voltage in the circuit is important; too much voltage may cause the circuit to blow the LED.
Investigating Questions
To further review the concepts in this activity and assess A team understanding, ask A Team the following questions:- What is the difference between a series and parallel circuit? (Answer: A series circuit has its parts connected in succession. A parallel circuit has its parts connected like a ladder. In a series circuit, current has only one path to flow through and therefore does not split. Thus, the current through each component in a series circuit is equal. The total voltage in a series circuit is equal to the sum of the voltage across each component in the circuit. In a parallel circuit, a ladder-like arrangement, current splits through the multiple pathways. Thus the sum of the current through each component is equal to the total current in the parallel circuit. Also, in a parallel circuit, the voltage across each component is equal. Each LED acts as a resistor in our fruit circuits. If the lights are in series, each LED gets the full current flow. When the LEDs are in parallel, the current is split among the different pathways, and thus each light has a smaller current as compared to each light in a series circuit.)
- What is a diode? (Answer: A diode is a device that contains a negative [anode] and a positive [cathode] component. Because of the positive and negative sides, a diode only conducts current in one direction. An LED is an example of a diode that is used in this experiment.)
- Have the A team calculate the total amount of energy wasted by transformers in the entire country. There are 100 million households in America. If each household wastes 25 watts on these transformers, that is 2.5 billion watts. At 10 cents a kilowatt-hour, that is 2,500,000,000 watts/1000 watts or $250,000 every hour. That is $2,190,000,000 ($2 billion) wasted every year.
Have a team try the activity again using different fruits or vegetables! Many fruits and vegetables work, such as lemons, limes, apples and carrots. Have A team compare and contrast the performance of different fruits and vegetables.
Have A team complete the activity again using an electrolyte solution, such as salt water or vinegar. Have them compare and contrast the performance of different electrolyte solutions.
To add a math component, have students use the multimeter to compare the flow of electricity for several different fruits as well as to the total amount of fruits used. Ask them to graph the results and hypothesize what is happening.
Activity Scaling
Have more advanced A team experiment with parallel and series configurations using different numbers of potatoes.XXX . XXX 4% zero Fruit + Electronics = Piano
The human body is electrically conductive. A piece of fruit will also conduct electricity, as will basically anything else that’s organic. We can leverage this fact to create a fun little afternoon project: a digital fruit piano. No soldering is necessary, and the whole thing takes less than an hour, even for a total beginner like my 9-year-old daughter. What sound does a banana make? Let’s fine out.
While humans and fruit do conduct electricity, they’re pretty bad at it. Both typically have an electrical resistance that’s in the 1-megaohm range, depending on how moist your skin or nectarine is. This design uses your body and the fruit as part of the circuit, flowing current through the human-fruit “wire”, but the high resistance means that the currents involved are tiny. The piano player isn’t going to feel a shock, or even feel anything at all. She’ll just lightly touch different bits of fruit to play a song, almost as if by magic.
This isn’t my original design. The idea of controlling a digital device by using the human body as part of the circuit has been around for quite a while, you can build a similar device yourself with only an Arduino and some hookup wire, a few resistors, an audio speaker, and a selection of bananas, pears, and peaches.
Tidak ada komentar:
Posting Komentar