


An electronic system must have an automated and integrated system in the sense of energy efficient and raw materials in a controlled working system safe and comfortable in the use and process of work and performance. many electronic components are used as controlling devices such as SCR (silicon control rectifier), TRIAC and DIAC, even 1010 or 2 code coding technique techniques (1 and 0) are used to control a work system of electronics that is widely applied both to the field of finance, automotive, industrial and transportation traffic as well as the pharmaceutical industry and avionics which is an industry that will trending into the future. if we look at the depths of human progress or slow down in the pattern of his situation moves for it needs to be developed with the help of industrial robotics on the development of pharmaceuticals and avionics as well as the processing of the quality and use of future energy energy into orbit orbit outside the earth.
comparative study on the automotive industry for Electronic stability control
Electronic stability control (ESC), also referred to as electronic stability program (ESP) or dynamic stability control (DSC), is a computerized technology that improves a vehicle's stability by detecting and reducing loss of traction (skidding). When ESC detects loss of steering control, it automatically applies the brakes to help "steer" the vehicle where the driver intends to go. Braking is automatically applied to wheels individually, such as the outer front wheel to counter oversteer or the inner rear wheel to counter understeer. Some ESC systems also reduce engine power until control is regained. ESC does not improve a vehicle's cornering performance; instead, it helps to minimize the loss of control.
Introduction
In 1995, three automobile manufacturers introduced ESC systems. Mercedes-Benz, supplied by Bosch, was the first to implement ESP with their Mercedes-Benz S 600 Coupé. That same year BMW, supplied by Bosch and ITT Automotive (later acquired by Continental Automotive Systems) introduced the system on the BMW 7 Series (E38) (DSC III)
Toyota's Vehicle Stability Control (VSC) system (also in 2004, a preventive system called Vehicle Dynamics Integrated Management (VDIM) appeared on the Toyota Crown Majesta in 1995.
In 1997, Audi introduced the first series production ESP for all-wheel drive vehicles (Audi A8 and Audi A6 with quattro (four-wheel drive system)). In 1998, Volvo Cars began to offer their version of ESC called Dynamic Stability and Traction Control (DSTC) on the new Volvo S80. Meanwhile, others investigated and developed their own systems.
During a moose test (swerving to avoid an obstacle), which became famous in Germany as "the elk test", the Swedish journalist Robert Collin of Teknikens Värld (World of Technology) in October 1997 rolled a Mercedes A-Class (without ESC) at 78 km/h. Because Mercedes-Benz promotes a reputation for safety, they recalled and retrofitted 130,000 A-Class cars with ESC. This produced a significant reduction in crashes and the number of vehicles with ESC rose. The availability of ESC in small cars like the A-Class ignited a market trend thus ESC became available for all models at least as an option.
General Motors (GM) worked with Delphi Automotive and introduced its version of ESC called "StabiliTrak" in 1997 for select Cadillac models. StabiliTrak was made standard equipment on all GM SUVs and vans sold in the U.S. and Canada by 2007 except for certain commercial and fleet vehicles. While the "StabiliTrak" name is used on most General Motors vehicles for the U.S. market, the "Electronic Stability Control" identity is used for GM overseas brands, such as Opel, Holden and Saab, except in the case of Saab's 9-7X which also uses the "StabiliTrak" name. The same year, Cadillac introduced an integrated vehicle handling and software control system, called Integrated Chassis Control System (ICCS), on the Cadillac Eldorado. It involves an omnibus computer integration of engine, traction control, Stabilitrak electronic stability control, steering, and adaptive continuously variable road sensing suspension (CVRSS), with the intent of improving responsiveness to driver input, performance, and overall safety, similar to Toyota/LexusVehicle Dynamics Integrated Management.
Ford's version of ESC, called AdvanceTrac, was launched in the year 2000. Ford later added Roll Stability Control to AdvanceTrac which was first introduced in Volvo XC90 in 2003 when Volvo Cars was fully owned by Ford and it is now being implemented in many Ford vehicles.
Operation
During normal driving, ESC works in the background, continuously monitoring steering and vehicle direction. It compares the driver's intended direction (determined through the measured steering wheel angle) to the vehicle's actual direction (determined through measured lateral acceleration, vehicle rotation (yaw), and individual road wheel speeds).
ESC intervenes only when it detects a probable loss of steering control, i.e. when the vehicle is not going where the driver is steering. This may happen, for example, when skidding during emergency evasive swerves, understeer or oversteer during poorly judged turns on slippery roads, or hydroplaning. During high-performance driving, ESC can intervene when unwanted, because steering input may not always be indicative of the intended direction of travel (i.e. controlled drifting). ESC estimates the direction of the skid, and then applies the brakes to individual wheels asymmetrically in order to create torque about the vehicle's vertical axis, opposing the skid and bringing the vehicle back in line with the driver's commanded direction. Additionally, the system may reduce engine power or operate the transmission to slow the vehicle down.
ESC can work on any surface, from dry pavement to frozen lakes. It reacts to and corrects skidding much faster and more effectively than the typical human driver, often before the driver is even aware of any imminent loss of control.This has led to some concern that ESC could allow drivers to become overconfident in their vehicle's handling and/or their own driving skills. For this reason, ESC systems typically alert the driver when they intervene, so that the driver knows that the vehicle's handling limits have been reached. Most activate a dashboard indicator light and/or alert tone; some intentionally allow the vehicle's corrected course to deviate very slightly from the driver-commanded direction, even if it is possible to more precisely match it.
All ESC manufacturers emphasize that the system is not a performance enhancement nor a replacement for safe driving practices, but rather a safety technology to assist the driver in recovering from dangerous situations. ESC does not increase traction, so it does not enable faster cornering (although it can facilitate better-controlled cornering). More generally, ESC works within the limits of the vehicle's handling and available traction between the tyres and road. A reckless maneuver can still exceed these limits, resulting in loss of control. For example, during hydroplaning, the wheels that ESC would use to correct a skid may lose contact with the road surface, reducing its effectiveness.
In July 2004, on the, Crown Majesta, Toyota offered a Vehicle Dynamics Integrated Management (VDIM) system that incorporated formerly independent systems, including ESC. This worked not only after the skid was detected but also to prevent the skid from occurring in the first place. Using electric variable gear ratio steering power steering, this more advanced system could also alter steering gear ratios and steering torque levels to assist the driver in evasive manoeuvres.
Due to the fact that stability control can be incompatible with high-performance driving (i.e. when the driver intentionally loses traction as in drifting), many vehicles have an over-ride control which allows the system to be partially or fully shut off. In simple systems, a single button may disable all features, while more complicated setups may have a multi-position switch or may never be turned fully off.
Effectiveness
Numerous studies around the world confirm that ESC is highly effective in helping the driver maintain control of the car, thereby saving lives and reducing the severity of crashes.
Components and design
ESC incorporates yaw rate control into the anti-lock braking system (ABS). Yaw is a rotation around the vertical axis; i.e. spinning left or right. Anti-lock brakes enable ESC to brake individual wheels. Many ESC systems also incorporate a traction control system (TCS or ASR), which senses drive-wheel slip under acceleration and individually brakes the slipping wheel or wheels and/or reduces excess engine power until control is regained. However, ESC serves a different purpose from that of ABS or Traction Control.[29]
The ESC system uses several sensors to determine what the driver wants (input). Other sensors indicate the actual state of the vehicle (response). The control algorithm compares driver input to vehicle response and decides, when necessary, to apply brakes and/or reduce throttle by the amounts calculated through the state space (set of equations used to model the dynamics of the vehicle).[43] The ESC controller can also receive data from and issue commands to other controllers on the vehicle such as an all wheel drive system or an active suspension system to improve vehicle stability and controllability.
The sensors used for ESC have to send data at all times in order to detect possible defects as soon as possible. They have to be resistant to possible forms of interference (rain, holes in the road, etc.). The most important sensors are:
- Steering wheel angle sensor: determines the driver's intended rotation; i.e. where the driver wants to steer. This kind of sensor is often based on AMR-elements.
- Yaw rate sensor: measures the rotation rate of the car; i.e. how much the car is actually turning. The data from the yaw sensor is compared with the data from the steering wheel angle sensor to determine regulating action.
- Lateral acceleration sensor: often an accelerometer
- Wheel speed sensor: measures the wheel speed.
Other sensors can include:
- Longitudinal acceleration sensor: similar to the lateral acceleration sensor in design, but can offer additional information about road pitch and also provide another source of vehicle acceleration and speed.
- Roll rate sensor: similar to the yaw rate sensor in design but improves the fidelity of the controller's vehicle model and correct for errors when estimating vehicle behavior from the other sensors alone.
ESC uses a hydraulic modulator to assure that each wheel receives the correct brake force. A similar modulator is used in ABS. ABS needs to reduce pressure during braking, only. ESC additionally needs to increase pressure in certain situations and an active vacuum brake booster unit may be utilized in addition to the hydraulic pump to meet these demanding pressure gradients.
The brain of the ESC system is the electronic control unit (ECU). The various control techniques are embedded in it. Often, the same ECU is used for diverse systems at the same time (ABS, Traction control system, climate control, etc.). The input signals are sent through the input-circuit to the digital controller. The desired vehicle state is determined based upon the steering wheel angle, its gradient and the wheel speed. Simultaneously, the yaw sensor measures the actual state. The controller computes the needed brake or acceleration force for each wheel and directs via the driver circuits the valves of the hydraulic modulator. Via a Controller Area Network interface the ECU is connected with other systems (ABS, etc.) in order to avoid giving contradictory commands.
Many ESC systems have an "off" override switch so the driver can disable ESC, which may be desirable when badly stuck in mud or snow, or driving on a beach, or if using a smaller-sized spare tire which would interfere with the sensors. Some systems also offer an additional mode with raised thresholds so that a driver can utilize the limits of adhesion with less electronic intervention. However, ESC defaults to "On" when the ignition is restarted. Some ESC systems that lack an "off switch", such as on many recent Toyota and Lexus vehicles, can be temporarily disabled through an undocumented series of brake pedal and handbrake operations. Furthermore, unplugging a wheel speed sensor is another method of disabling most ESC systems. The ESC implementation on newer Ford vehicles cannot be completely disabled even through the use of the "off switch". The ESC will automatically reactivate at highway speeds, and below that if it detects a skid with the brake pedal depressed.
Future
The market for ESC is growing quickly, especially in European countries such as Sweden, Denmark, and Germany. For example, in 2003 in Sweden the purchase rate on new cars with ESC was 15%. The Swedish road safety administration issued a strong ESC recommendation and in September 2004, 16 months later, the purchase rate was 58%. A stronger ESC recommendation was then given and in December 2004, the purchase rate on new cars had reached 69% and by 2008 it had grown to 96%. ESC advocates around the world are promoting increased ESC use through legislation and public awareness campaigns and by 2012, most new vehicles should be equipped with ESC.
Just as ESC is founded on the Anti-lock braking system (ABS), ESC is the foundation for new advances such as Roll Stability Control or Active rollover protection that works in the vertical plane much like ESC works in the horizontal plane. When RSC detects impending rollover (usually on transport trucks or SUVs), RSC applies brakes, reduces throttle, induces understeer, and/or slows down the vehicle.
The computing power of ESC facilitates the networking of active and passive safety systems, addressing other causes of crashes. For example, sensors may detect when a vehicle is following too closely and slow down the vehicle, straighten up seat backs, and tighten seat belts, avoiding and/or preparing for a crash.
Electronic stability control also works with the vehicle’s traction control. This electronic system measures how fast the wheels are turning. If one is spinning faster, which can indicate it’s on a slippery surface, the traction control system will activate the brake on that wheel, and may also momentarily reduce the engine’s power, helping the spinning tire to regain its grip. (Sometimes you want a bit of wheel spin, such as when you’re trying to get out of deep snow, which is why it’s recommended that you turn off the traction control if you’re stuck.)
Both ABS and traction control are primarily meant for going forward. If things start to go sideways, that’s when ESC comes in. It depends primarily on three sensors, which measure yaw, wheel speed, and steering angle.
The yaw sensor is located at the centre of the vehicle and, as the name suggests, measures yaw – how much the vehicle is moving to the left or right of its vertical axis. The system then compares this to the steering angle – the direction of the front wheels, and how far you have them turned. If you’ve turned the wheels to the left, and the car is obediently turning in that direction, then all is good.
But if the sensors realize you have your wheels turned to the left, but the car is heading to the right, it knows you’re skidding sideways. To help get you out of it, it uses some or all of the components from the ABS and traction control systems, including braking specific wheels and reducing engine power to bring everything back in line with the direction of the wheels, and get the driver back in control of the vehicle. It works when either the front or the rear wheels lose traction and the vehicle starts to skid. It continually monitors the vehicle, in all weather conditions, and automatically engages as required. It is possible to temporarily disable it on a few models, but will come back on as the default the next time the engine is started.
The system is very efficient, and Transport Canada’s studies suggest a 29 per cent reduction in crashes caused by drivers’ loss of control because of it. But there’s only so much it can do, and you can only argue so far with the laws of physics. To help ensure safe driving, be sure your tires are in good condition, with enough tread and properly inflated. Make highway lane changes gradually, rather than wrenching the wheel to get over. Accelerate slowly and steadily, rather than hitting the throttle hard, especially in slippery conditions. And if you get into gravel or a soft surface, such as on the road shoulder, don’t hit the brakes. Instead, slowly ease off the throttle, and gradually steer back onto the pavement.


XX00 _____ XXX Helicopter flight controls
A helicopter pilot manipulates the helicopter flight controls to achieve and maintain controlled aerodynamic flight.[1] Changes to the aircraft flight control system transmit mechanically to the rotor, producing aerodynamic effects on the rotor blades that make the helicopter move in a deliberate way. To tilt forward and back (pitch) or sideways (roll), requires that the controls alter the angle of attack of the main rotor blades cyclically during rotation, creating differing amounts of lift (force) at different points in the cycle. To increase or decrease overall lift requires that the controls alter the angle of attack for all blades collectively by equal amounts at the same time, resulting in ascent, descent, acceleration and deceleration.
A typical helicopter has three flight control inputs—the cyclic stick, the collective lever, and the anti-torque pedals. Depending on the complexity of the helicopter, the cyclic and collective may be linked together by a mixing unit, a mechanical or hydraulic device that combines the inputs from both and then sends along the "mixed" input to the control surfaces to achieve the desired result. The manual throttle may also be considered a flight control because it is needed to maintain rotor speed on smaller helicopters without governors. The governors also help the pilot control the collective pitch on the helicopter's main rotors, to keep a stable, more accurate flight.

Location of flight controls in a helicopter
Controls
Cyclic
The cyclic control, commonly called the cyclic stick or just cyclic, is similar in appearance on most helicopters to a joystick from a conventional aircraft. It is usually located between the pilot's legs, but on some helicopters, such as the Robinson R22, it is a central pillar that either pilot can manipulate.
The control is called the cyclic because it changes the pitch angle of the rotor blades cyclically. That is, the pitch, or feathering angle, of the rotor blades changes depending upon their position as they rotate around the hub, so that all blades have the same incidence at the same point in the cycle. The change in cyclic pitch has the effect of changing the angle of attack of the blades, and thus the lift generated by a single blade as it moves around the rotor disk. This in turn causes the blades to fly up or down in sequence, depending on the changes in lift affecting each individual blade.
The result is to tilt the rotor disk in a particular direction, resulting in the helicopter moving in that direction. If the pilot pushes the cyclic forward, the rotor disk tilts forward, and the rotor produces a thrust vector in the forward direction. If the pilot pushes the cyclic to the right, the rotor disk tilts to the right and produces thrust in that direction, causing the helicopter to move sideways in a hover or to roll into a right turn during forward flight, much as in a fixed-wing aircraft.
Any rotor system has a delay between the point in rotation where the controls introduce a change in pitch and the point where the desired change in the rotor blade's flight occurs. This difference is caused by phase lag, often confused with gyroscopic precession. A rotor is an oscillatory system that obeys the laws that govern vibration—which, depending on the rotor system, may resemble the behaviour of a gyroscope.
Collective
The collective pitch control, or collective lever, is normally located on the left side of the pilot's seat with an adjustable friction control to prevent inadvertent movement. The collective changes the pitch angle of all the main rotor blades collectively (i.e., all at the same time) and independent of their position. Therefore, if a collective input is made, all the blades change equally, and as a result,the helicopter increases or decreases its total lift derived from the rotor. In level flight this would cause a climb or descent, while with the helicopter pitched forward an increase in total lift would produce an acceleration together with a given amount of ascent.
The collective pitch control in a Boeing CH-47 Chinook is called a thrust control, but serves the same purpose, except that it controls two rotor systems, applying differential collective pitch.
Anti-torque pedals
The anti-torque pedals are located in the same place as the rudder pedals in an airplane, and serve a similar purpose—they control the direction that the nose of the aircraft points. Applying the pedal in a given direction changes the tail rotor blade pitch, increasing or reducing tail rotor thrust and making the nose yaw in the direction of the applied pedal.
Throttle
Helicopter rotors are designed to operate at a specific rotational speed. The throttle controls the power of the engine, which is connected to the rotor by a transmission. The throttle setting must maintain enough engine power to keep the rotor speed within the limits where the rotor produces enough lift for flight. In many helicopters, the throttle control is a single or dual motorcycle-style twist grip mounted on the collective control (rotation is opposite of a motorcycle throttle), while some multi-engine helicopters have power levers.
In many piston engine-powered helicopters, the pilot manipulates the throttle to maintain rotor speed. Turbine engine helicopters, and some piston helicopters, use governors or other electro-mechanical control systems to maintain rotor speed and relieve the pilot of routine responsibility for that task. (There is normally also a manual reversion available in the event of a governor failure.)
Name | Directly controls | Primary effect | Secondary effect | Used in forward flight | Used in hover flight |
---|---|---|---|---|---|
Cyclic (longitudinal) | Varies main rotor blade pitch with fore and aft movement | Tilts main rotor disk forward and back via the swashplate | Induces pitch nose down or up | To adjust forward speed and control rolled-turn | To move forwards/backwards |
Cyclic (lateral) | Varies main rotor blade pitch with left and right movement | Tilts main rotor disk left and right through the swashplate | Induces roll in direction moved | To create movement to sides | To move sideways |
Collective | Collective angle of attack for the rotor main blades via the swashplate | Increase/decrease pitch angle of all main rotor blades equally, causing the aircraft to ascend/descend | Increase/decrease torque. Note: in some helicopters the throttle control(s) is a part of the collective stick. Rotor speed is kept basically constant throughout the flight. | To adjust power through rotor blade pitch setting | To adjust skid height/vertical speed |
Anti-torque pedals | Collective pitch supplied to tail rotor blades | Yaw rate | Increase/decrease torque and engine speed (less than collective) | To adjust sideslip angle | To control yaw rate/heading |
Flight conditions
There are three basic flight conditions for a helicopter: hover, forward flight and autorotation.
Hover
Some pilots consider hovering the most challenging aspect of helicopter flight. This is because helicopters are generally dynamically unstable, meaning that deviations from a given attitude are not corrected without pilot input. Thus, frequent control inputs and corrections must be made by the pilot to keep the helicopter at a desired location and altitude. The pilot's use of control inputs in a hover is as follows: the cyclic is used to eliminate drift in the horizontal plane, (e.g., forward, aft, and side to side motion); the collective is used to maintain desired altitude; and the tail rotor (or anti-torque system) pedals are used to control nose direction or heading. It is the interaction of these controls that can make learning to hover difficult, since often an adjustment in any one control requires the adjustment of the other two, necessitating pilot familiarity with the coupling of control inputs needed to produce smooth flight.
Forward flight
In forward flight, a helicopter's flight controls behave more like those in a fixed-wing aircraft. Moving the cyclic forward makes the nose pitch down, thus losing altitude and increasing airspeed. Moving the cyclic back makes the nose pitch up, slowing the helicopter and making it climb. Increasing collective (power) while maintaining a constant airspeed induces a climb, while decreasing collective (power) makes the helicopter descend. Coordinating these two inputs, down collective plus aft (back) cyclic or up collective plus forward cyclic causes airspeed changes while maintaining a constant altitude. The pedals serve the same function in both a helicopter and an airplane, to maintain balanced flight. This is done by applying a pedal input in the direction necessary to center the ball in the turn and bank indicator.
Autorotation
Differential pitch control
For helicopters with two horizontally-mounted rotors, changes in attitude often require having each rotor behave inversely in response to the standard control inputs from the pilot. Those with coaxial rotors (like a Kamov Ka-50) have both rotors mounted on the same mast, one above the other on concentric drive shafts contra-rotating–spinning in opposite directions on a shared axis–and make yaw changes by increasing the collective pitch of the rotor spinning in the direction of the desired turn while simultaneously reducing the collective pitch of the other, creating dissymettry of torque.
Tandem-rotor craft (like a Boeing CH-47 Chinook) also employ two rotors spinning in opposite directions–termed counter-rotation when it occurs from two separate points on the same airframe–but have the rotors on separate drive shafts through masts at the nose and tail. This configuration uses differential collective pitch to change the overall pitch attitude of the aircraft. When the pilot moves the cyclic forward to pitch the nose down and accelerate forward, the helicopter responds by decreasing collective pitch on the front rotor and increases collective pitch on the rear rotor proportionally, pivoting the two ends around their common center of mass. Changes in yaw are made with differential cyclic pitch, the front rotor altering cyclic pitch in the direction desired and the opposite pitch applied to the rear, once again pivoting the craft around its center.
Conversely, the synchropter and transverse-mounted rotor counter rotating rotorcraft have two large horizontal rotor assemblies mounted side by side, (like a Bell/Boeing V-22 tilt rotor) helicopters use differential collective pitch to affect the roll of the aircraft. Like tandem rotors, differential cyclic pitch is used to control movement about the yaw axis.
How Helicopters Work
In addition to moving up and down, helicopters can fly forward, backward and sideways. This kind of directional flight is achieved by tilting the swash plate assembly with the cyclic, which alters the pitch of each blade as it rotates. As a result, every blade produces maximum lift at a particular point. The rotor still generates lift, but it also creates thrust in the direction that the swash plate assembly is tilted. This causes the helicopter to lean -- and fly -- in a certain direction. The pilot can impart additional directional control by depressing or easing up on the foot pedals, which increases or decreases the counteracting thrust of the tail rotor.
Let's assume for a moment that the helicopter we discussed in the last section needs to fly forward. This is the pilot's procedure:
- First, he or she nudges the cyclic lever forward.
- That input is transmitted to the lower swash plate and then to the upper swash plate.
- The swash plates tilt forward at an amount equal to the input.
- The rotor blades are pitched lower in the front of the rotor assembly than behind it.
- This increases the angle of attack -- and creates lift -- at the back of the helicopter.
- The unbalanced lift causes the helicopter to tip forward and move in that direction.
When the aircraft reaches about 15 to 20 knots of forward airspeed, it begins to transition from hovering flight to full forward flight. At this point, known as effective translational lift, or ETL, the pilot eases up on the left foot pedal and moves closer to a neutral setting. He or she also feels a shudder in the rotor system as the helicopter begins to fly out of rotor wash (the turbulence created by a helicopter's rotor) and into clean air. In response, the rotor will try to lift up and slow the aircraft automatically. To compensate, the pilot will continue to push the cyclic forward to keep the helicopter flying in that direction with increasing airspeed.
A helicopter that is flying forward can stop in mid-air and begin hovering very quickly. We'll cover this signature maneuver next.
The defining characteristic of a helicopter is its ability to hover at any point during a flight. To achieve hovering, a pilot must maintain the aircraft in nearly motionless flight over a reference point at a constant altitude and on a heading (the direction that the front of the helicopter is pointing). This may sound easy, but it requires tremendous experience and skill.
Before we tackle the technique of hovering, let's take a moment to discuss nap-of-the-earth (NOE) flight, another unique characteristic of helicopters. NOE flight describes a helicopter located just above the ground or any obstacles on the ground. Military pilots perfected the technique during Vietnam as a means to become more elusive to ground-based weapons. In fact, film footage from the era often shows helicopters rapidly skimming the Earth's surface, machine-gunners firing from open rear doors or hovering with their skids just a few feet off the ground as troops disembark at a target location.
Of course, any helicopter taking off or landing must undertake NOE flight, if only for a few moments. It's a particularly critical time for a helicopter because a wild attitude adjustment could tip the craft too far and bring the rotor blades in contact with an obstacle. Attitude, for our purposes, refers to the helicopter's orientation in relation to the helicopter's direction of motion. You'll also hear flight-minded folks talk about attitude in reference to an axis, such as the horizon.
With that said, here's the basic technique to bring a helicopter into a hovering position:
- First, the pilot must cease any directional flying. For example, if flying the helicopter forward, the pilot must ease back on the cyclic until the helicopter's forward motion stops and the aircraft remains motionless over a point on the ground.
- Next, it's important that the pilot can detect small changes in the aircraft's altitude or attitude. He or she accomplishes this by locating a fixed point outside the cockpit and tracking how the helicopter moves relative to that point.
- Finally, the pilot adjusts the collective to maintain a fixed altitude and adjusts the foot pedals to maintain the direction that the helicopter is pointing.
To maintain a stabilized hover, the pilot must make small, smooth, coordinated corrections on all of the controls. In fact, one of the most common errors of novice pilots is to overcompensate while trying to hover. For example, if the helicopter begins to move rearward, the pilot must be careful not to apply too much forward pressure on the cyclic because the aircraft will not just come to a stop but will start drifting forward.
Over the years, innovations in helicopter design have made the machines safer, more reliable and easier to control. The next page presents a few of these innovations to provide a glimpse of how far helicopters have come and where they might go in the future.
The modern helicopter, like any complex machine, is an accumulation of innovations from numerous inventors and engineers. Some of these modifications improve performance significantly without changing the overall appearance of the aircraft. For example, Arthur Young's stabilizer bar looks small and insignificant when compared to the gross anatomy of a chopper, but it revolutionized vertical-lift flight. Other innovations are less subtle and seem to give the helicopter a complete makeover. Let's check out a few changes.
One significant advancement in the last decade has been the no-tail rotor, or NOTAR, helicopter. As you now know, vertical-lift flight is impossible without a tail rotor to counteract the torque produced by the main rotor. Unfortunately, the much-smaller tail rotor makes a lot of noise and is often easily damaged. The NOTAR helicopter solves both of these problems. Here's how it works: A large fan at the rear of the fuselage blows spent air from the main rotor down the tail boom. Slots along the side of the tail boom and at the end of the boom allow this air to escape. This creates a sideways force that counteracts the main rotor's torque. Varying the amount of air expelled from the rear slot provides additional directional control.
Some helicopters started receiving a second engine, which can operate the main rotor if the main engine fails. For example, the UH-60 Black Hawk helicopter, the workhorse of the U.S. Army, features this design improvement. Either engine can keep the aircraft aloft on its own, enabling the pilot to land safely in the event of an emergency.
Scientists have also fiddled with the main rotor assembly in an attempt to simplify one of the most complex parts of a helicopter. In the late 1990s, researchers developed a solid-state adaptive rotor system incorporating piezoelectric sheets. A piezoelectric material is one in which its molecules bend and twist in response to an electric field. In a rotor assembly, piezoelectric sheets -- not mechanical linkages -- twist sections of the blade root, thereby changing the pitch of the blades as they rotate. This eliminates parts in the rotor hub and decreases the chance of a mechanical failure.
Finally, it's worth mentioning those strange machines, known as tiltrotors, that bring together the best features of helicopters and airplanes. A tiltrotor aircraft takes off like a helicopter, with its two main rotors upright. But when it's airborne, the pilot can tip the rotors forward 90 degrees, enabling the machine to fly like conventional turboprop airplane. The V-22 Osprey, which completed a successful test flight in 1989, operates in this fashion.
None of these innovations has made helicopters less absurd-looking. Some, like the tiltrotor, only increase the aircraft's awkward visual appearance.

XX00_____ XXX 10001 + 10 Gear Bearings and Gear-Bearing Transmissions
Gear bearings are conceptual mechanical components so named because they function as gears and as roller and/or thrust bearings. Gear bearings will be essential components of the next generation of compact, large-mechanical- advantage gear drives.

Figure 1 shows a working gear-bearing transmission with 70/1 gain in a 1.25-in.-diameter-by-0.75-in. (3.18-cm-diameter-by-1.91-cm) package. Gear bearings exist as spur- or helical-gear variants of two basic types: roller gear bearings and phase-shifted gear bearings. For the sake of brevity, only spur-gear variants are described here; suffice it to say that the principles of operation of the helicalgear variants are similar.

Figure 2(a) depicts selected aspects of roller spur-gear bearings and a partial planetary assembly containing roller spurgear bearings. The diameter of the roller portion of each roller spur-gear bearing equals the pitch diameter of the spur-gear portion. The gear teeth are crowned at the roller end, such that the apogee of the crown on each tooth lies at the roller diameter/ pitch diameter: This arrangement provides thrust-bearing strength as well as matching of speeds and, hence, efficiency.
The planetary assembly would inherently hold itself together, without bracing by other structures. For example, if a planetary roller gear bearing shown in the cross-sectional view were pushed downward, the axial sliding of its teeth with respect to the ring- and sun-gear teeth is stopped by the abutment of the planetary roller against the crowns of the ring-gear teeth. When the same planetary gear is pushed upward, its axial sliding is blocked by abutment of the planetary teeth against the ring roller. Similar interactions prevent axial sliding of the sun roller gear bearing beyond the limits imposed by tooth/roller contacts between the sun and planetary roller gear bearings.
An additional advantage is that by enforcing the desired relative locations of gears more precisely, the incorporation of the rollers increases (relative to simple spur gears) the accuracy of meshing of spur-gear teeth. At the same time, the gears act as highly efficient and precise cages and carriers for the rollers. The net result is a superior, simpler, and relatively inexpensive assembly.
Figure 2(b) presents a simple example of a phase-shifted spur-gear bearing. The teeth in the upper and lower halves are shifted angularly, relative to each other, by precisely a half-tooth interval. This gear bearing meshes with a copy of itself. The upper and lower teeth are beveled and partly interdigitated where they meet. The contact between the beveled surfaces of the upper and lower teeth provide a thrust-bearing capability in a manner similar to that of the contact between the crowns and rollers of the roller gear bearings. Moreover, a planetary assembly containing phaseshifted gears holds itself together in a manner similar to that of an assembly containing roller gear bearings as described above.
XX00 ___ XXX 10001 + 10 = >2 dan <5 DIAC and TRIAC on Electronic Control – Working and Characteristics
The DIode AC switch, or Diac for short, is another solid state, three-layer, two-junction semiconductor device but unlike the transistor the Diac has no base connection making it a two terminal device, labelled A1 and A2.
Diac’s are an electronic component which offer no control or amplification but act much like a bidirectional switching diode as they can conduct current from either polarity of a suitable AC voltage supply.
In our tutorial about SCR’s and Triacs, we saw that in ON-OFF switching applications, these devices could be triggered by simple circuits producing steady state gate currents as shown.

When switch, S1 is open no gate current flows and the lamp is “OFF”. When switch S1 is closed, gate current IGflows and the SCR conducts on the positive half cycles only as it is operating in quadrant Ι.
We remember also that once gated “ON”, the SCR will only switch “OFF” again when its supply voltage falls to a values such that its Anode current, IA is less than the value of its holding current, IH.
If we wish to control the mean value of the lamp current, rather than just switch it “ON” or “OFF”, we could apply a short pulse of gate current at a pre-set trigger point to allow conduction of the SCR to occur over part of the half-cycle only. Then the mean value of the lamp current would be varied by changing the delay time, T between the start of the cycle and the trigger point. This method is known commonly as “phase control”.
But to achieve phase control, two things are needed. One is a variable phase shift circuit (usually an RC passive circuit), and two, some form of trigger circuit or device that can produce the required gate pulse when the delayed waveform reaches a certain level. One such solid state semiconductor device that is designed to produce these gate pulses is the Diac.
The diac is constructed like a transistor but has no base connection allowing it to be connected into a circuit in either polarity. Diacs are primarily used as trigger devices in phase-triggering and variable power control applications because a diac helps provide a sharper and more instant trigger pulse (as opposed to a steadily rising ramp voltage) which is used to turn “ON” the main switching device.
The diac symbol and the voltage-current characteristics curves of the diac are given below.
Diac Symbol and I-V Characteristics

We can see from the above diac I-V characteristics curves that the diac blocks the flow of current in both directions until the applied voltage is greater than VBR, at which point breakdown of the device occurs and the diac conducts heavily in a similar way to the zener diode passing a sudden pulse of voltage. This VBR point is called the Diacs breakdown voltage or breakover voltage.
In an ordinary zener diode the voltage across it would remain constant as the current increased. However, in the diac the transistor action causes the voltage to reduce as the current increases. Once in the conducting state, the resistance of the diac falls to a very low value allowing a relatively large value of current to flow. For most commonly available diacs such as the ST2 or DB3, their breakdown voltage typically ranges from about ±25 to 35 volts. Higher breakover voltage ratings are available, for example 40 volts for the DB4 diac.
This action gives the diac the characteristic of a negative resistance as shown above. As the diac is a symmetrical device, it therefore has the same characteristic for both positive and negative voltages and it is this negative resistance action that makes the Diac suitable as a triggering device for SCR’s or triacs.
Diac Applications
As stated above, the diac is commonly used as a solid state triggering device for other semiconductor switching devices, mainly SCR’s and triacs. Triacs are widely used in applications such as lamp dimmers and motor speed controllers and as such the diac is used in conjunction with the triac to provide full-wave control of the AC supply as shown.
Diac AC Phase Control

As the AC supply voltage increases at the beginning of the cycle, capacitor, C is charged through the series combination of the fixed resistor, R1 and the potentiometer, VR1 and the voltage across its plates increases. When the charging voltage reaches the breakover voltage of the diac (about 30 V for the ST2), the diac breaks down and the capacitor discharges through the diac.
The discharge produces a sudden pulse of current, which fires the triac into conduction. The phase angle at which the triac is triggered can be varied using VR1, which controls the charging rate of the capacitor. Resistor, R1 limits the gate current to a safe value when VR1 is at its minimum.
Once the triac has been fired into conduction, it is maintained in its “ON” state by the load current flowing through it, while the voltage across the resistor–capacitor combination is limited by the “ON” voltage of the triac and is maintained until the end of the present half-cycle of the AC supply.
At the end of the half cycle the supply voltage falls to zero, reducing the current through the triac below its holding current, IH turning it “OFF” and the diac stops conduction. The supply voltage then enters its next half-cycle, the capacitor voltage again begins to rise (this time in the opposite direction) and the cycle of firing the triac repeats over again.
Triac Conduction Waveform

Then we have seen that the Diac is a very useful device which can be used to trigger triacs and because of its negative resistance characteristics this allows it to switch “ON” rapidly once a certain applied voltage level is reached. However, this means that whenever we want to use a triac for AC power control we will need a separate diac as well. Fortunately for us, some bright spark somewhere replaced the individual diac and triac with a single switching device called a Quadrac.
The Quadrac
The Quadrac is basically a Diac and Triac fabricated together within a single semiconductor package and as such are also known as “internally triggered triacs”. This all in one bi-directional device is gate controlled using either polarity of the main terminal voltage which means it can be used in full-wave phase-control applications such as heater controls, lamp dimmers, and AC motor speed control, etc.

Like the triac, quadracs are a three-terminal semiconductor switching device labelled MT2 for main terminal one (usually the anode), MT1 for main terminal two (usually the cathode) and G for the gate terminal.
The quadrac is available in a variety of package types depending upon their voltage and current switching requirements with the TO-220 package being the most common as it is designed to be an exact replacement for most triac devices.
Diac Summary
In this diac we have seen that the diac such as the ST2 or DB3 is a two-terminal voltage blocking device that can conduct in either direction. Diacs posses negative resistance characteristics which allows them to switch “ON” rapidly once a certain applied voltage level is reached.
Since the diac is a bidirectional device, when paired with the BTAxx-600A or IRT80 series of switching triacs it makes it useful as a triggering device in phase control and general AC circuits such as light dimmers and motor speed controls.
Quadracs are simply triacs with an internally connected diac. As with triacs, quadracs are bidirectional AC switches which are gate controlled for either polarity of main terminal voltage.
There are several applications where it is preferred to regulate the power fed to a load. For instance: using electrical methods controlling the speed of a motor or fan. But, these methods do not allow a fine control over the flow of power in a system additionally; there is an extensive wastage of power. In the present days, such devices have been developed which can allow a fine control over the flow of large blocks of power in a system. These devices perform as controlled switches and can complete the duties of controlled rectification, regulation and inversion of power in a load. The essential semiconductor switching devices are UJT, SCR, DIAC and TRIAC.
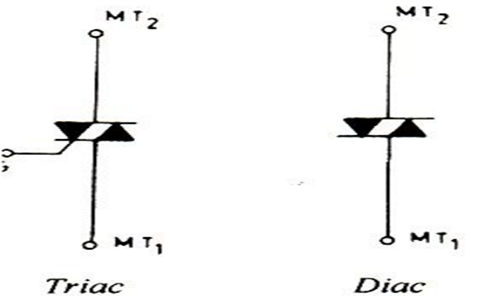
Earlier we have studied about the basic electrical and electronic components such as transistor, capacitor, diode, etc. But, to understand the switching devices like scr, diac and triac we have to know about the thyristor. A thyristor is a one type of semiconductor device includes three or more terminals. It is a unidirectional similar to a diode but switched like a transistor. Thyristors are used to control high voltages and currents in the motors, heating and lighting applications.
Difference between Diac and Triac
The differences between diac and triac mainly include what are a diac and triac, construction of triac and diac, working, characteristics and applications.
What are Diac and Triac?
We know that, thyristor is a half wave device like a diode and that will supply only half power. A Triac device comprises of two thyristors that are connected in opposite direction but in parallel but, it is controlled by the same gate. Triac is a 2-dimensional thyristor which is activated on both halves of the i/p AC cycle using + Ve or -Ve gate pulses. The three terminals of the Triac are MT1; MT2 & gate terminal (G). Generating pulses are applied between MT1 and gate terminals. The ‘G’ current to switch 100A from triac is not more than 50mA or so.
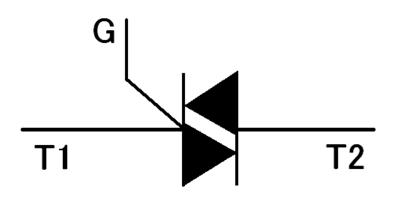
The DIAC is a bi-directional semiconductor switch that can be switched on in both polarities. The full form of the name DIAC is diode alternating current. Diac is connected back to back using two zener diodes and the main application of this DIAC is, it is widely used to help even activating of a TRIAC when used in AC switches, dimmer applications and starter circuits for florescent lamps.

Construction and Operation of DIAC
Basically, the DIAC is a two terminal device; it is a combination of parallel semiconductor layers that allows activating in one direction.This device is used to activating device for the triac. The basic construction of diac consist of two terminals namely MT1 and MT2. When the MT1 terminal is designed +Ve with respect to the terminal MT2, the transmission will take place to the p-n-p-n structure that is another four layer diaode. The diac can be performing for both the direction. Then symbol of the diac look like a transistor.

The DIAC is basically a diode that conducts after a ‘break-over’ voltage, selected VBO, and is exceeded. When the diode surpasses the break-over voltage, then it goes into the negative dynamic resistance of region. This causes in a reduce in the voltage drop across the diode with rising voltage. So there is a quick increase in the current level that is mannered by the device.
The diode leftovers in its transmission state until the current through it falls below, what is termed the holding current, which is usually chosen by the letters IH. The holding current, the DIAC reverts to its non-conducting state. Its behavior is bidirectional and thus its function takes place on both halves of an alternating cycle.
Characteristics of DIAC
V-I characteristics of a diac is shown below
Volt-ampere characteristic of a diac is shown in figure. Its looks like a letter Z due to symmetrical switching characteristics for each polarity of the applied voltage.
The diac performs like an open-circuit until its switching is exceeded. At that position the diac performs until its current decreases toward zero. Because of its abnormal construction, doesn’t switch sharply into a low voltage condition at a low current level like the triac or SCR, once it goes into transmission, the diac preserves an almost continuous –Ve resistance characteristic, that means, voltage reduces with the enlarge in current. This means that, unlike the triac and the SCR, the diac cannot be estimated to maintain a low voltage drop until its current falls below the level of holding current.

Construction and Operation of TRIAC
Traic is a three terminal device and the terminals of the triac are MT1, MT2 and Gate. Here the gate terminal is the control terminal. The flow of current in the triac is bi directional that means current can flow in both the directions. The structure of triac is shown in the below figure. Here, in the structure of triac, two SCRs are connected in the anti parallel and it will acts like a switch for both the directions. In the above structure, the MT1 and gate terminals are near to each other. When the gate terminal is open, the triac will obstruct the both the polarities of the voltage across the MT1 & MT2.

To know more about TRIAC please follow the below link: TRIAC – Definition, Applications & Working
Characteristics of TRIAC
The V-I characteristics of TRIAC are discussed below
The triac is designed with two SCRs which are fabricated in the opposite direction in a crystal. Operating characteristics of triac in the 1st and 3rd quadrants are similar but for the direction of flow of current and applied voltage.
The V-I characteristics of triac in the first and third quadrants are basically equal to those of an SCR in the first quadrant.
It can be functioned with either +Ve or –Ve gate control voltage but in typical operation generally the gate voltage is +Ve in first quadrant and -Ve in third quadrant.
The supply voltage of the triac to switch ON depends upon the gate current. This allows utilizing a triac to regulate AC power in a load from zero to full power in a smooth and permanent manner with no loss in the device control.

Thus, this is all about the difference between diac and triac, working and its characteristics
Switch mode power supply ( SMPS ) for efficiency energy
Linear voltage regulators are generally much more efficient and easier to use than equivalent voltage regulator circuits made from discrete components such a zener diode and a resistor, or transistors and even op-amps.
The most popular linear and fixed output voltage regulator types are by far the 78… positive output voltage series, and the 79… negative output voltage series. These two types of complementary voltage regulators produce a precise and stable voltage output ranging from about 5 volts up to about 24 volts for use in many electronic circuits.
There is a wide range of these three-terminal fixed voltage regulators available each with its own built-in voltage regulation and current limiting circuits. This allows us to create a whole host of different power supply rails and outputs, either single or dual supply, suitable for most electronic circuits and applications. There are even variable voltage linear regulators available as well providing an output voltage which is continually variable from just above zero to a few volts below its maximum voltage output.
Most d.c. power supplies comprise of a large and heavy step-down mains transformer, diode rectification, either full-wave or half-wave, a filter circuit to remove any ripple content from the rectified d.c. producing a suitably smooth d.c. voltage, and some form of voltage regulator or stabiliser circuit, either linear or switching to ensure the correct regulation of the power supplies output voltage under varying load conditions. Then a typical d.c. power supply would look something like this:
Typical DC Power Supply

These typical power supply designs contain a large mains transformer (which also provides isolation between the input and output) and a dissipative series regulator circuit. The regulator circuit could consist of a single zener diode or a three-terminal linear series regulator to produce the required output voltage. The advantage of a linear regulator is that the power supply circuit only needs an input capacitor, output capacitor and some feedback resistors to set the output voltage.
Linear voltage regulators produce a regulated DC output by placing a continuously conducting transistor in series between the input and the output operating it in its linear region (hence the name) of its current-voltage (i-v) characteristics. Thus the transistor acts more like a variable resistance which continually adjusts itself to whatever value is needed to maintain the correct output voltage. Consider this simple series pass transistor regulator circuit below:
Series Transistor Regulator Circuit

Here this simple emitter-follower regulator circuit consists of a single NPN transistor and a DC biasing voltage to set the required output voltage. As an emitter follower circuit has unity voltage gain, applying a suitable biasing voltage to the transistors base, a stabilised output is obtained from the emitter terminal.
Since a transistor provides current gain, the output load current will be much higher than the base current and higher still if a Darlington transistor arrangement is used.
Also, providing that the input voltage is sufficiently high enough to get the desired output voltage, the output voltage is controlled by the transistors base voltage and in this example is given as 5.7 volts to produce a 5 volt output to the load as approximately 0.7 volts is dropped across the transistor between the base and emitter terminals. Then depending upon the value of the base voltage, any value of emitter output voltage can be obtained.
While this simple series regulator circuit will work, the downside to this is that the series transistor is continually biased in its linear region dissipating power in the form of heat as a result of its V*I product, since all the load current must pass through the series transistor, resulting in poor efficiency, wasted power and continuous heat generation.
Also, one of the disadvantages that series voltage regulators have is that, their maximum continuous output current rating is limited to just a few amperes or so, so are generally used in applications where low power outputs are required. When higher output voltage or current power supplies are required, the normal practice is to use a switching regulator commonly known as a switch-mode power supply to convert the mains voltage into whatever higher power output is required.
Switch Mode Power Supplies, or SMPS, are becoming common place and have replaced in most cases the traditional linear ac-to-dc power supplies as a way to cut power consumption, reduce heat dissipation, as well as size and weight. Switch-mode power supplies can now be found in most PC’s, power amplifiers, TV’s, dc motor drives, etc., and just about anything that requires a highly efficient supply as switch-mode power supplies are increasingly becoming a much more mature technology.
By definition, a switch mode power supply (SMPS) is a type of power supply that uses semiconductor switching techniques, rather than standard linear methods to provide the required output voltage. The basic switching converter consists of a power switching stage and a control circuit. The power switching stage performs the power conversion from the circuits input voltage, VIN to its output voltage, VOUT which includes output filtering.
The major advantage of the switch mode power supply is its higher efficiency, compared to standard linear regulators, and this is achieved by internally switching a transistor (or power MOSFET) between its “ON” state (saturated) and its “OFF” state (cut-off), both of which produces lower power dissipation. This means that when the switching transistor is fully “ON” and conducting current, the voltage drop across it is at its minimal value, and when the transistor is fully “OFF” there is no current flow through it. So the transistor is acting like an ideal switch.
As a result, unlike linear regulators which only offer step-down voltage regulation, a switch mode power supply, can offer step-down, step-up and negation of the input voltage using one or more of the three basic switch mode circuit topologies: Buck, Boost and Buck-Boost. This refers to how the transistor switch, inductor, and smoothing capacitor are connected within the basic circuit.
Buck Switch Mode Power Supply
The Buck switching regulator is a type of switch mode power supply circuit that is designed to efficiently reduce DC voltage from a higher voltage to a lower one, that is it subtracts or “Bucks” the supply voltage, thereby reducing the voltage available at the output terminals without changing the polarity. In other words, the buck switching regulator is a step-down regulator circuit, so for example a buck converter can convert say, +12 volts to +5 volts.
The buck switching regulator is a DC-to-DC converter and one of the simplest and most popular type of switching regulator. When used within a switch mode power supply configuration, the buck switching regulator uses a series transistor or power MOSFET (ideally an insulated gate bipolar transistor, or IGBT) as its main switching device as shown below.
The Buck Switching Regulator

We can see that the basic circuit configuration for a buck converter is a series transistor switch, TR1 with an associated drive circuit that keeps the output voltage as close to the desired level as possible, a diode, D1, an inductor, L1 and a smoothing capacitor, C1. The buck converter has two operating modes, depending on if the switching transistor TR1 is turned “ON” or “OFF”.
When the transistor is biased “ON” (switch closed), diode D1 becomes reverse biased and the input voltage, VIN causes a current to flow through the inductor to the connected load at the output, charging up the capacitor, C1. As a changing current flows through the inductor coil, it produces a back-emf which opposes the flow of current, according to Faraday’s law, until it reaches a steady state creating a magnetic field around the inductor, L1. This situation continues indefinitely as long as TR1 is closed.
When transistor TR1 is turned “OFF” (switch open) by the controlling circuitry, the input voltage is instantly disconnected from the emitter circuit causing the magnetic field around the inductor to collapse inducing a reverse voltage across the inductor. This reverse voltage causes the diode to become forward biased, so the stored energy in the inductors magnetic field forces current to continue to flow through the load in the same direction, and return back through diode.
Then the inductor, L1 returns its stored energy back to the load acting like a source and supplying current until all the inductor’s energy is returned to the circuit or until the transistor switch closes again, whichever comes first. At the same time the capacitor also discharges supplying current to the load. The combination of the inductor and capacitor forms an LC filter smoothing out any ripple created by the switching action of the transistor.
Therefore, when the transistor solid state switch is closed, current is supplied from the supply, and when the transistor switch is open, current is supplied by the inductor. Note that the current flowing through the inductor is always in the same direction, either directly from the supply or via the diode but obviously at different times within the switching cycle.
As the transistor switch is being continuously closed and opened, the average output voltage value will therefore be related to the duty cycle, D which is defined as the conduction time of the transistor switch during one full switching cycle. If VIN is the supply voltage, and the “ON” and “OFF” times for the transistor switch are defined as: tON and tOFF, then the output voltage VOUT is given as:
Buck Converter Duty Cycle

The buck converters duty cycle can also be defined as:

So the larger the duty cycle, the higher the average DC output voltage from the switch mode power supply. From this we can also see that the output voltage will always be lower than the input voltage since the duty cycle, D can never reach one (unity) resulting in a step-down voltage regulator. Voltage regulation is obtained by varying the duty cycle and with high switching speeds, up to 200kHz, smaller components can be used thereby greatly reducing a switch mode power supply’s size and weight.
Another advantage of the buck converter is that the inductor-capacitor (LC) arrangement provides very good filtering of the inductor current. Ideally the buck converter should be operated in a continuous switching mode so that the inductor current never falls to zero. With ideal components, that is zero voltage drop and switching losses in the “ON” state, the ideal buck converter could have efficiencies as high as 100%.
As well as the step-down buck switching regulator for the basic design of a switch mode power supply, there is another operation of the fundamental switching regulator that acts as a step-up voltage regulator called the Boost Converter.
Boost Switch Mode Power Supply
The Boost switching regulator is another type of switch mode power supply circuit. It has the same types of components as the previous buck converter, but this time in different positions. The boost converter is designed to increase a DC voltage from a lower voltage to a higher one, that is it adds too or “Boosts” the supply voltage, thereby increasing the available voltage at the output terminals without changing the polarity. In other words, the boost switching regulator is a step-up regulator circuit, so for example a boost converter can convert say, +5 volts to +12 volts.
We saw previously that the buck switching regulator uses a series switching transistor within its basic design. The difference with the design of the boost switching regulator is that it uses a parallel connected switching transistor to control the output voltage from the switch mode power supply. As the transistor switch is effectively connected in parallel with the output, electrical energy only passes through the inductor to the load when the transistor is biased “OFF” (switch open) as shown.
The Boost Switching Regulator

In the Boost Converter circuit, when the transistor switch is fully-on, electrical energy from the supply, VIN passes through the inductor and transistor switch and back to the supply. As a result, none of it passes to the output as the saturated transistor switch effectively creates a short-circuit to the output. This increases the current flowing through the inductor as it has a shorter inner path to travel back to the supply. Meanwhile, diode D1becomes reverse biased as its anode is connected to ground via the transistor switch with the voltage level on the output remaining fairly constant as the capacitor starts to discharge through the load.
When the transistor is switched fully-off, the input supply is now connected to the output via the series connected inductor and diode. As the inductor field decreases the induced energy stored in the inductor is pushed to the output by VIN, through the now forward biased diode. The result of all this is that the induced voltage across the inductor L1reverses and adds to the voltage of the input supply increasing the total output voltage as it now becomes, VIN + VL.
Current from the smoothing capacitor, C1 which was used to supply the load when the transistor switch was closed, is now returned to the capacitor by the input supply via the diode. Then the current supplied to the capacitor is the diode current, which will always be ON or OFF as the diode is continually switched between forward and reverse status by the switching actions of transistor. Then the smoothing capacitor must be sufficiently large enough to produce a smooth steady output.
As the induced voltage across the inductor L1 is negative, it adds to the source voltage, VINforcing the inductor current into the load. The boost converters steady state output voltage is given by:

As with the previous buck converter, the output voltage from the boost converter depends upon the input voltage and duty cycle. Therefore, by controlling the duty cycle, output regulation is achieved. Not also that this equation is independent of the value of the inductor, the load current, and the output capacitor.
We have seen above that the basic operation of a non-isolated switch mode power supply circuit can use either a buck converter or boost converter configuration depending upon whether we require a step-down (buck) or step-up (boost) output voltage. While buck converters may be the more common SMPS switching configuration, boost converters are commonly used in capacitive circuit applications such as battery chargers, photo-flashes, strobe flashes, etc, because the capacitor supplies all of the load current while the switch is closed.
But we can also combine these two basic switching topologies into a single non-isolating switching regulator circuit called unsurprisingly, a Buck-Boost Converter.
Buck-Boost Switching Regulator
The Buck-Boost switching regulator is a combination of the buck converter and the boost converter that produces an inverted (negative) output voltage which can be greater or less than the input voltage based on the duty cycle. The buck-boost converter is a variation of the boost converter circuit in which the inverting converter only delivers the energy stored by the inductor, L1, into the load. The basic buck-boost switch mode power supply circuit is given below.
The Buck-Boost Switching Regulator

When the transistor switch, TR1, is switched fully-on (closed), the voltage across the inductor is equal to the supply voltage so the inductor stores energy from the input supply. No current is delivered to the connected load at the output because diode, D1, is reverse biased. When the transistor switch is fully-off (open), the diode becomes forward biased and the energy previously stored in the inductor is transferred to the load.
In other words, when the switch is “ON”, energy is delivered into the inductor by the DC supply (via the switch), and none to the output, and when the switch is “OFF”, the voltage across the inductor reverses as the inductor now becomes a source of energy so the energy stored previously in the inductor is switched to the output (through the diode), and none comes directly from the input DC source. So the voltage dropped across the load when the switching transistor is “OFF” is equal to the inductor voltage.
The result is that the magnitude of the inverted output voltage can be greater or smaller (or equal to) the magnitude of the input voltage based on the duty cycle. For example, a positive-to-negative buck-boost converter can convert 5 volts to 12 volts (step-up) or 12 volts to 5 volts (step-down).
The buck-boost switching regulators steady state output voltage, VOUT is given as:

Then the buck-boost regulator gets its name from producing an output voltage that can be higher (like a boost power stage) or lower (like a buck power stage) in magnitude than the input voltage. However, the output voltage is opposite in polarity from the input voltage.
Switch Mode Power Supply Summary
The modern switch mode power supply, or SMPS, uses solid-state switches to convert an unregulated DC input voltage to a regulated and smooth DC output voltage at different voltage levels. The input supply can be a true DC voltage from a battery or solar panel, or a rectified DC voltage from an AC supply using a diode bridge along with some additional capacitive filtering.
In many power control applications, the power transistor, MOSFET or IGFET, is operated in its switching mode were it is repeatedly turned “ON” and “OFF” at high speed. The main advantage of this is that the power efficiency of the regulator can be quite high because the transistor is either fully-on and conducting (saturated) or full-off (cut-off).
There are several types of DC-to-DC converter (as opposed to a DC-to-AC converter which is an inverter) configurations available, with the three basic switching power supply topologies looked at here being the Buck, Boost, and the Buck-Boost switching regulators. All three of these topologies are non-isolated, that is their input and output voltages share a common ground line.
Each switching regulator design has its own unique properties with regards to the steady-state duty cycles, relationship between the input and output current, and the output voltage ripple produced by the solid-state switch action. Another important property of these switch mode power supply topologies is the frequency response of the switching action to the output voltage.
Regulation of the output voltage is achieved by the percentage control of the time that the switching transistor is in the “ON” state compared to the total ON/OFF time. This ratio is called the duty cycle and by varying the duty cycle, (D the magnitude of the output voltage, VOUT can be controlled.
The use of a single inductor and diode as well as fast switching solid-state switches capable of operating at switching frequencies in the kilohertz range, within the switch mode power supply design, allows for the size and weight of the power supply to be greatly reduced. This is because there would be no large and heavy step-down (or step-up) voltage mains transformers within their design. However, if isolation is required between the input and output terminals, a transformer must be included before the converter.
The two most popular non-isolated switching configurations are the buck (subtractive) and the boost (additive) converters.
The buck converter is a type of switch-mode power supply that is designed to convert electrical energy from one voltage to a lower one. The buck converter operates with a series connected switching transistor. As the duty cycle, D < 1, the output voltage of the buck is always smaller than the input voltage, VIN.
The boost converter is a type of switch-mode power supply that is designed to convert electrical energy from one voltage to a higher one. The boost converter operates with a parallel connected switching transistor which results in a direct current path between VINand VOUT via the inductor, L1 and diode, D1. This means there is no protection against short-circuits on the output.
By varying the duty cycle, (D) of a boost converter, the output voltage can be controlled and with D < 1, the DC output from the boost converter is greater than input voltage VINas a consequence of the inductors self-induced voltage.
Also, the output smoothing capacitors in Switch-mode Power Supplies is assumed to be very large, which results in a constant output voltage from the switch mode supply during the transistors switching action.
++++++++++++++++++++++++++++++++++++++++++++++++++++++++++++++++++++++++++++++

+++++++++++++++++++++++++++++++++++++++++++++++++++++++++++++++++++++++++++++++
100% Guarantee of JOB after Two months industrial automation training program includes from basic to advance level modules designed for both students and working professionals. Our experts with hands-on experience on the technology and working experience makes sure to deliver the best to you. Call @91-9953489987, 9711287737.
BalasHapus