D I G I T A L = Direct Gyroscope To Alignment
1001 ( 2 - an )

Picture of GPS satellites in orbit

Global positioning system is a system for determining the location on the earth's surface with the aid of alignment (synchronization) satellite signals at each singular point on earth. The system uses 24 satellites that transmit microwave signals to Earth. This signal is received by a receiver on the surface, and is used to determine the location, speed, direction, and time. A similar system with GPS, among others, the Russian GLONASS, Galileo European Union, IRNSS India.
The system was developed by the US Department of Defense, the full name is NAVSTAR GPS (common mistake is that the NAVSTAR is an acronym, this is wrong, NAVSTAR is the name given by John Walsh, a determinant of important policy in the GPS program). This set of satellites maintained by the 50th Space Wing United States Air Force. This system maintenance costs approximately US $ 750 million per year, including the replacement of old satellites, and research and development.
GPS Tracker or often called GPS Tracking is a technology AVL (Automated Vehicle Locater) which allows users to track the position of the vehicle, fleet or the car in Real-Time. GPS Tracking utilizing a combination of GSM and GPS technology to determine the coordinates of an object, and then translate it in the form of a digital map ( digital = direct gyroscope to alignment ) .
Ways of working
Positioning Satellite
Gravimetric Datum ORIENTATION.SVG
NMEA 2000 Network Installation with GPS
This system uses a number of satellites that are in orbit of the earth, which transmit their signals to earth and captured by a receiver. There are three important parts of this system, the control section, part of space, and part of the user.
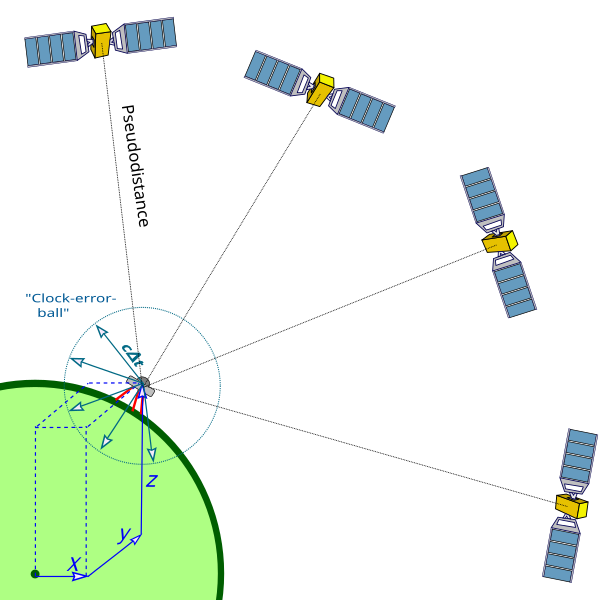
Satellite Positioning
control section
As the name suggests, this section to control. Each satellite can be a bit outside the orbit, so this section is to track the satellite's orbit, location, altitude, and speed. The signals from the satellite is received by the control section, corrected, and sent back to the satellite. Correction of the data the precise location of the satellite is called ephemeris data, which will send it to our navigation tool.part sky
This section consists of a collection of satellites that are in earth orbit, about 12,000 miles above the Earth's surface. Set of these satellites are arranged so that the navigation tool at any time can receive signals from at least four satellites. The satellite signals can pass through clouds, glass, or plastic, but can not pass through buildings or mountains. The satellite has an atomic clock, and will also emit information 'time / h' is. This data is transmitted by the code 'pseudo-random'. Each satellite has its own code. The code number will usually be displayed in the navigation tool, then we can identify the satellite signal is being received by the tool. This data is useful for a navigation tool to measure the distance between the satellite navigation tool, which will be used to measure the coordinates of the location. Satellite signal strength will also help tool in the calculation. The signal strength is more influenced by the location of the satellite, an instrument will receive stronger signals from satellites that are right on top of it (think satellite locations such as the position of the sun when 12 noon) compared with satellites that are in the line of the horizon (think satellite locations such as the position of the sunset / published).
There are two types of waves that are currently used for satellite-based navigation tool in general, the former is better known as L1 at 1575.42 MHz. The L1 signal to be received by the navigation tool. Satellites also issued L2 wave at a frequency of 1227.6 MHz. L2 wave is used for military purposes and not for the public.

Users section
This section consists of navigation tools used. The satellite will transmit the almanac data and ephemeris to be received by the navigation tool on a regular basis. Almanac data contains the approximate location (approximate location) satellites continuously emitted by the satellite. Ephemeris data transmitted by the satellite, and is valid for about 4-6 hours. To show the coordinates of a point (two-dimensional), navigation tools need at least a signal from three satellites. To show the elevation data of a point (three-dimensional), required additional satellite signals from one piece again.
From the signals emitted by the satellite collection, navigation tools will do the calculations, and the end result is the coordinate position of the tool. The more number of satellite signals received by a tool, will make the tool calculate the coordinates of its position more precisely.The accuracy of GPS navigation tool
Accuracy or precision of a concern for determining the coordinates of a point / location. This position coordinates will always have 'error factor', which is better known as the 'degree of accuracy'. For example, the tool shows a coordinate point with an accuracy of 3 meters, which means that the actual position can be anywhere within a radius of 3 meters from the point of coordinates (location) is. The smaller the number of accuracy (meaning accuracy is higher), its position will become increasingly precise tools. Price tool will also increase along with the increase in the level of accuracy that can be achieved.
In everyday use, the accuracy is more often influenced by factors around which reduces the strength of the satellite signal. Because the satellite signal can not penetrate solid objects well, so when using the tool, it is important to take notice of sky that can be seen.
Explanation of the satellite signal to geography
When the tool is at deep valleys (eg, accuracy of 15 meters), then the accuracy rate will be much lower than in the pasture (eg, accuracy of 3 meters). In the meadow or mountain peak, the number of satellites that can be reached by the tool will be much more than from a mountain valley. So, do not expect to use this navigational tool in a cave.
Because of this navigation tool relies fully on the satellite, the satellite signal becomes very important. This satellite-based navigation tool may not work optimally when there is interference on satellite signals. There are many things that can reduce the strength of the satellite signal:
Geographical conditions, as described above. As long as we can still see
sky is wide enough, the tool is still able to function.
Forest. The more forested, hence diminishing the signal can be received.
Water. Do not expect to use these tools when diving.
Car window film, especially those containing metal.
Electronic equipment can emit electromagnetic waves.
Buildings. Not only when in the building, located between 2 pieces of high-rise building will also cause such effects were in the valley.
Signals that bounce, for example when you are in between tall buildings, can disrupt calculations navigation tool navigation tool that can show the wrong position or inaccurate.
Explanation of the display screen of the GPS satellite signals.
The number of satellites and their signal strength that can be accessed by means of navigation can be seen on the device screen. Nearly all satellite-based navigation tool can display data about a satellite that is connected to the appliance, satellite locations, and signal strength.

NMEA 2000 Network Installation with GPS
There are two types of built-in antenna navigation tools most commonly encountered, namely types and Quad Helix Patch. Type Patch, flattened shape while quad helix shaped like a tube. Of course both have their advantages and disadvantages of each. In everyday use, many factors that affect its function. A navigation tool that has the patch antenna, the better the signal reception when the tool is held horizontally parallel to the earth. While the device has a quad helix antenna, will be better when held upright, the upper part towards the sky. To make sure, check the antenna specifications navigation tools.
In everyday use, it is often necessary external antenna, for example, consumption in the four-wheeled vehicles. There are several types of external antennas that can be selected. Keep in mind that not all types of navigation tool has a slot for an external antenna.Active external antenna
Called active because it is equipped with a Low Noise Amplifier (LNA), signal boosters, because the signal is reduced when crossing the wires. That is, this type require a power source to perform its function, which is usually taken from a navigation tool. Navigation tools so the battery will drain faster. The advantage, the cable can be used longer than the passive type.Passive external antenna
Because it is not supplemented by a signal amplifier, the batteries do not run out quickly. But the cables used can not be all the active type.Eksternal re-radiating antenna
This type consists of two parts, the first catch satellite signals, the latter emits a signal. Because the signal is emitted, then this type does not require a cable connection to a navigation tool. Navigation tools will receive signals as usual. Of course, this kind require an additional power source, but instead of a navigation tool used. For the type of navigation tool that does not have a slot for an external antenna, this type is a good alternative than having to modify the navigation tool.= Antenna Combo
This type of antenna is a merger between the antenna for navigation devices and mobile phones. The power source is required for its use.
Coordinates are displayed by means of navigation are the coordinates of the position of the external antenna. So the placement of the external antenna is also noteworthy.
DGPS
DGPS (Differential Global Positioning System) is a system or a way to improve GPS, using ground stations, which emit location corrections. With this system, then when a navigation tool accept correction and put it into the calculations, the accuracy of the navigation tools will increase. Therefore using ground stations, the signal can not cover a large area.
Although differ in how things work, SBAS (Satellite Based Augmentation System) in general can be said is DGPS using satellites. Coverage area is much broader than the DGPS that uses ground stations. There are several SBAS which is known, that is WAAS (Wide Area Augmentation System), EGNOS (European Geostationary Navigation Overlay Service), and MSAS (Multi-functional Satellite Augmentation System). Is managed by the US WAAS, EGNOS by the European Union, and MSAS by Japan. The three systems are mutually compatible with one another, meaning that a navigation tool that can use one system, will be able to use two other systems as well. At this time only WAAS already fully operational and can be enjoyed by users of the navigation tools in the world. However, a DGPS with ground stations are functioning properly, can improve the accuracy exceeds / is equal to the increase that can be achieved by SBAS.
In general, can be divided into two major parts, namely "real time (live)" and "Post processing (after the end of the action)". The purpose of the 'real time' is a navigation tool that uses SBAS or DGPS signals directly during use. While the 'post processing' means that the data collected by means of navigation in the process of re-using data from ground stations DGPS. There are many DGPS ground stations around the world that we can use for this, both free and paid versions, even we can directly use it over the internet.
Although DGPS or SBAS can improve accuracy, but on condition that the transmitted signal contains a correction for the area in which we use the navigation tool. If it does not contain the corrected data for the region, there will be an increase accuracy.
Some sense of the termCold & Warm start
In detail specification navigation tools, usually written, the time required for cold and warm start. When the navigation apparatus is switched off, the appliance is still storing satellite data that have locked before. One of the stored data is ephemeris data, and the data is still valid for about 4-6 hours (for easier, use the reference time of 4 hours).
When switched on again, the tool will search for the satellite navigation based on the data stores. When the data is still stored within this time, then the existing data can still be used by satellite navigation tool to lock and cause faster navigation tool to lock the satellite. This is called "Warm start".
When the stored data has expired, meaning that exceeds the period above, the navigation apparatus can not wear it. So the navigation tools have to start the whole process from the beginning, and the lead time required becomes longer. This is called "Cold Start". This whole process only lasts a few minutes.
DGPS (Differential Global Positioning System) is a system or a way to improve GPS, using ground stations, which emit location corrections. With this system, then when a navigation tool accept correction and put it into the calculations, the accuracy of the navigation tools will increase. Therefore using ground stations, the signal can not cover a large area.
Although differ in how things work, SBAS (Satellite Based Augmentation System) in general can be said is DGPS using satellites. Coverage area is much broader than the DGPS that uses ground stations. There are several SBAS which is known, that is WAAS (Wide Area Augmentation System), EGNOS (European Geostationary Navigation Overlay Service), and MSAS (Multi-functional Satellite Augmentation System). Is managed by the US WAAS, EGNOS by the European Union, and MSAS by Japan. The three systems are mutually compatible with one another, meaning that a navigation tool that can use one system, will be able to use two other systems as well. At this time only WAAS already fully operational and can be enjoyed by users of the navigation tools in the world. However, a DGPS with ground stations are functioning properly, can improve the accuracy exceeds / is equal to the increase that can be achieved by SBAS.
In general, can be divided into two major parts, namely "real time (live)" and "Post processing (after the end of the action)". The purpose of the 'real time' is a navigation tool that uses SBAS or DGPS signals directly during use. While the 'post processing' means that the data collected by means of navigation in the process of re-using data from ground stations DGPS. There are many DGPS ground stations around the world that we can use for this, both free and paid versions, even we can directly use it over the internet.
Although DGPS or SBAS can improve accuracy, but on condition that the transmitted signal contains a correction for the area in which we use the navigation tool. If it does not contain the corrected data for the region, there will be an increase accuracy.
Some sense of the termCold & Warm start
In detail specification navigation tools, usually written, the time required for cold and warm start. When the navigation apparatus is switched off, the appliance is still storing satellite data that have locked before. One of the stored data is ephemeris data, and the data is still valid for about 4-6 hours (for easier, use the reference time of 4 hours).
When switched on again, the tool will search for the satellite navigation based on the data stores. When the data is still stored within this time, then the existing data can still be used by satellite navigation tool to lock and cause faster navigation tool to lock the satellite. This is called "Warm start".
When the stored data has expired, meaning that exceeds the period above, the navigation apparatus can not wear it. So the navigation tools have to start the whole process from the beginning, and the lead time required becomes longer. This is called "Cold Start". This whole process only lasts a few minutes.
IPX7 waterproof
These standards established by the IEC (International Electrotechnical Commission), the first number describes testing the resilience of the tool against solid objects, and the second number describes the resistance to liquid (water). When the tool has only been tested against one state (solid or liquid), then the letter 'X' is placed on the first or second number.
IP X7 meaning: X indicates the device was not tested against solid objects, while the number 7 means it can be soaked in water with a depth of 15 cm - 1 meter (on the Garmin website added: 30 minutes).RoHS version
In the manual satellite-based navigation tools, may be found to this specification. It is the provisions made by the European Union regarding the restriction of use of six types of hazardous materials in electronic equipment manufactured after July 1, 2006. RoHS stands for Restriction of use of certain Hazardous Substances. Six types of materials that are restricted are Cadmium (Cd), mercury / mercury (Hg), hexavalent chromium (Cr (VI)), polybrominated biphenyls (PBBs) and polybrominated diphenyl ethers (PBDEs) and lead / lead (Pb). All types of materials can affect people's health, including waste electronic equipment that we use.proposition 65
This is a provision made by the government of the state of California, USA. This provision aims to protect residents of california and drinking water sources from contamination of hazardous materials. Under this provision, every plant is required to include a warning on their products, so that users can make informed decisions to protect themselves.There are many materials that are considered hazardous, and these can change over time. A material is considered hazardous may be withdrawn from the list if later proved harmless.
The term comes from the word 'Geo' taken from the geography, and 'caching' is taken from the activities storing / hiding something. Geocaching is actually a game to find the 'treasure' hidden by using a satellite-based navigation tools.
Simple activities, first hide some small items (pens, pencils, etc.) at several separate places, such that it is not easily visible. Record the coordinates of each of these places. Then several groups trying to find all the hidden items. Certainly will not be too easy to find, because each tool has a different accuracy.
This activity can be combined with other activities, for example, activities to clean up the garden, or outbound, and so on.
Which stands for ' dillution of Precision ' , closely linked to a satellite location in the sky. DOP value obtained from mathematical calculations, which show the 'level of trust' calculation of a location. When satellites are located close together, then the DOP value will increase, causing the accuracy of satellite-based navigation tool is reduced. When satellites are located far apart, then the value of the DOP will be reduced so that more accurate navigational tool.
When the DOP value is smaller than 5 (some say under 4), then the accuracy will be obtained fairly accurate. There are some values are often encountered, namely HDOP (Horizontal dilution of Precision), VDOP (Vertical dilution of Precision), and PDOP (Positional dilution of Precision - a three-dimensional position).location coordinates
A point whose coordinates can be displayed in several formats. Each user can set this format in navigation tools, map source program, or other computer programs. This format can be set from the setting of each program / navigation tools.
There are several commonly used formats: hddd.ddddd0; hddd0mm, mmm '; hddd0mm'ss.s "; + Ddd, ddddd0. So that a point can be demonstrated in several ways, for example: point S6010.536 'E106049.614' equals E106.826910 S6.175600 point is equal to point S6010'32.2 "E106049'36.9" equals -6.175600 106.826910. The first part is the coordinates Latitude, followed by coordinates Longitude or often abbreviated as Lat / Long.
Stay-Up Method
Stay-Up method is an instance method or a combination of several methods that are used as a simplification in solving the problems faced by programmers. This method is suitable to seek a coordinated points at a location to base programming with components or GPS device and a gadget, in order to get the value of the distance between the two points, and if there is an object moving in a unit time to it can be determined the speed and acceleration , The combined method is adopted from land Navigation methods and methods Two Stay Two Stray, in order to get a description of methods of Stay-Up as follows:
Waiting latitude1-2 and longtitude1-2 data information (from GPS)
Encode data (GPS)
Finding the difference of the two data (distance)
Doing delays per-minute (speed)
Thus, in this method a distance indicator system (gadgets) works passively or immovable bed (Stay), which can only be operated or moved (up) if no data is sent by a source in this case the GPS device to a member of the gadget. Resources (GPS) provides certain coded data ( latitude - Longtitude ) which is then translated or encoded by a member (gadget) into an information that is easily understood and understandable for subsequent use as a reference determinant of an outcome, ie the distance and speed.POI tour guide
What is meant by this term is the combination of regular POI with images, text, voice, and proximity alarms. When a satellite-based navigation tools into the distance set on the alarm wherewith proximity of a POI, the satellite-based navigation tool will automatically display the photographs and text, and voice prompts. Tour guide plus a set of POI route that has been determined to be a tour guide during the trip. But if the map used are different, then the route indicated by navigation tool will likely be different. POI tourguide can only be enjoyed by users of satellite-based navigation tools specific Garmin product, the nuvi series that have MP3 capabilities, Zumo, street pilot c550, c580, 2730, 2820, 7200, 7500.
Stay-Up method is an instance method or a combination of several methods that are used as a simplification in solving the problems faced by programmers. This method is suitable to seek a coordinated points at a location to base programming with components or GPS device and a gadget, in order to get the value of the distance between the two points, and if there is an object moving in a unit time to it can be determined the speed and acceleration , The combined method is adopted from land Navigation methods and methods Two Stay Two Stray, in order to get a description of methods of Stay-Up as follows:
Waiting latitude1-2 and longtitude1-2 data information (from GPS)
Encode data (GPS)
Finding the difference of the two data (distance)
Doing delays per-minute (speed)
Thus, in this method a distance indicator system (gadgets) works passively or immovable bed (Stay), which can only be operated or moved (up) if no data is sent by a source in this case the GPS device to a member of the gadget. Resources (GPS) provides certain coded data ( latitude - Longtitude ) which is then translated or encoded by a member (gadget) into an information that is easily understood and understandable for subsequent use as a reference determinant of an outcome, ie the distance and speed.POI tour guide
What is meant by this term is the combination of regular POI with images, text, voice, and proximity alarms. When a satellite-based navigation tools into the distance set on the alarm wherewith proximity of a POI, the satellite-based navigation tool will automatically display the photographs and text, and voice prompts. Tour guide plus a set of POI route that has been determined to be a tour guide during the trip. But if the map used are different, then the route indicated by navigation tool will likely be different. POI tourguide can only be enjoyed by users of satellite-based navigation tools specific Garmin product, the nuvi series that have MP3 capabilities, Zumo, street pilot c550, c580, 2730, 2820, 7200, 7500.
usefulness
Military
GPS is used for the purposes of war, such as the lead toward the bomb, or know where the troops are. In this way we can find out where friends where the opponent to avoid any targets, or determine the movement of troops.
Navigation
GPS lot is also used as a navigation tool such as a compass. Some types of vehicles have been equipped with GPS for navigation tools, by adding a map, it can be used to guide the driver, so the driver can know which path should be selected to achieve the desired goal.
Geographic Information Systems
For the purposes of Geographic Information Systems, GPS is often also included in the mapping, such as measuring the distance of the border, or as a reference measurement.
Vehicle tracking system
Other uses are as a GPS vehicle tracker, with GPS for helping owner of the vehicle / fleet manager can know anywhere vehicle / asset moving in today.
earthquake monitoring
Even today, GPS with high accuracy can be used to monitor the movement of the ground, which order only mm a year. Monitoring is useful for estimating ground motion of earthquakes, both the movement of volcanic or tectonic
Construction of multi - storey buildingnamely by installing sensors in each elbow and the foundation of the building is monitored from the GPS to see the robustness and shapes correspond to the ideal state of a building project rise buildings (skyscrapers) desired usually use tool sensors, electronics and mechanics associated with GPS and projections of soil movement in the fore monitored with satellite.
Other satellite navigation system being developed by other countries are:
Beidou - the local system in the PRC which will be developed into an international system called COMPASS.
Galileo - System being developed by the European Union, with the help of China, Israel, India, Morocco, Saudi Arabia, South Korea, and Ukraine.
GLONASS - the Russian system is being repaired.
Indian Regional Navigational Satellite System (IRNSS) - The system developed India.
Beidou - the local system in the PRC which will be developed into an international system called COMPASS.
Galileo - System being developed by the European Union, with the help of China, Israel, India, Morocco, Saudi Arabia, South Korea, and Ukraine.
GLONASS - the Russian system is being repaired.
Indian Regional Navigational Satellite System (IRNSS) - The system developed India.
there is also a supplementary role of satellite-based navigation tool in the health world
The role of navigation tools in the public health world is inseparable from the use of GIS (Geographical Information System), or the term generally is mapping. When used in the medical field, these two things are closely related to health information systems in a broad sense.
Its use in the world of public health aims to help provide information to enable decision makers to do their work more easily and accurately. The decision makers here does not necessarily mean the administrative structure of governance, but also could mean communities and individuals. If the decision makers do not use the information provided, then this activity is a waste of time, effort, and funds.
Currently, many people who using means of satellite-based navigation and mapping in planning, deciding, implementing, and evaluating programs - community-based programs. The most often use is the Non Governmental Organization (NGOs), both international and national, in disaster control programs.
The biggest problem is the cost and the resources available, so rarely interested parties to develop it. If already available, the knowledge about the benefits of the information obtained is also still doubtful. The question that needs to be answered is: How important is the benefit gained? This question became central because even though information from the mapping is not available, all of the activities during this time remains to be done.
True, no information from the mapping, public health programs can do. But, what about the 'time' required to achieve the desired conditions? And whether it can be accelerated if the decision taken is the right target? Therein lies the main functions of the health information system, this system should be able to provide the necessary information so that decision makers can do a good job. The same mistake should not be repeated time when that will come. For example, an outbreak of the same disease is not resolved in the same way from year to year, so it ended up being a regular plague.
Mapping along with the use of satellite-based navigation tool is a part of the overall system health information. Without the support of the other parts, the benefits gained will not be maximized. Furthermore, if the decision made has nothing to do with the information obtained, the information systems function to be lost.
The type of information that can be displayed depending on the data entered into the system this mapping. The mapping system can combine the numeric data (in the form of statistics, survey results, monthly reports, and so on) from the health information system with visual map. So it can be seen at the macro and micro.
Coverage mapping does not need such a large area, but can be used for a small area, such as a village. The map in the above example also consist of a combination of areas that are smaller, which can be selected to be displayed on the screen. Types of visual information like the above are not absolute should be available, since the analysis can be performed using the figures contained in the health information system.
So, the main function of the above mapping is to facilitate decision-makers to improve the existing conditions. With the presence of visual information like this, then the user may be easier to look at the situation and conditions. The next step remains at decision makers.
X . I
How Global Positioning System (GPS) works?
After Effect - Satellite tracking
Introduction
Global Positioning System (GPS), has
found itself as a gateway for the cutting edge technology for this
century. It was designed and developed by USA during the late 19th
century after recognizing its importance in military applications. Due
to its accurate positioning capabilities and navigation and timing
services it was later open for civilian uses as well.
Working of GPS
A GPS system consists of
• A GPS enabled product like a GPS phone or a GPS Receiver
• A plan for the extent of
GPS data. Some plans provide all information’s about the locality you
are in. While other plans are used only to track your position.
• A service plan to provide the phone’s location.
• Satellites around the Earth which provides your exact position using “Trilateration”.
Basically a GPS receiver comprises of a
receiver, and the help of the 27 earth-orbiting satellites, which
rotates in the medium earth orbit. Out of these 27 satellites only 24
are in use and the rest is kept as backup for any failed satellite.
Suppose you want to know your exact position using GPS, the receiver
determines the exact locations of a minimum of 3 satellites above you
and also your position with respect to the satellite. Then a method
called “Trilateration” is used to find your exact location. These
satellites make two complete rotations around the Earth everyday. So, a
minimum of 4 satellites are assured to receive the GPS signals.
Trilateration Method
When the receiver sends the signal to
locate itself, it finds out three nearest satellites of known positions.
The receiver then calculates the distance between one satellite and the
receiver. If the distance is “X”, then it draws an imaginary sphere
with “X” as the radius from the receiver to the satellite and also the
satellite as the centre. Similarly, the same process is repeated for the
next two satellites. Thus three spheres are drawn with just two
possible positions. Out of these one point will be in space and the
other will be the location of the receiver. Thus the exact position of
the receiver is found out. Usually the receivers try to locate more than
four satellites so as to increase the accuracy of the location. The
Earth is made as the fourth sphere so that two points converge with the
imaginary spheres of the other three satellites. This method is commonly
called 3-D Trilateration method.
Just as phones use radio signals, the
satellites also use low power, high frequency radio signals so as to
compute the location of it with respect to the receiver. They are also
used to compute the distance between the device and the satellites. The
distance is calculated by knowing the time it took to reach the
receiver.
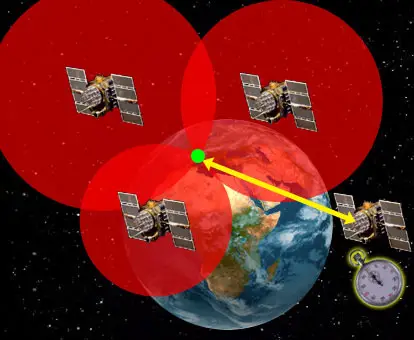
Trilateration Method
Methods for calculating Distance and Location of the Satellite and Receiver
As explained earlier, to find the distance and locations of the satellite and receiver, the parameter time is computed.
At a time ‘t’, a pseudo-random code is
transmitted from the satellite. At the same time the receiver also
starts running the same pseudo random digital pattern. But there will be
a time-lag, when the signals from the satellite reaches the receiver.
The signal’s travel time will be equal to the length of this delay. When
this time is multiplied by the speed of light, the distance between the
satellite and receiver is obtained.
For this method to work properly,
accurate clocks that can read a small time as nanosecond is required in
both the receiver and satellite. But these type of atomic clocks are
rather expensive and so cannot be bought by all types of consumers.
The only problem to this solution is to
use a quartz clock and an atomic clock. The atomic clock will be mounted
on the satellite. The quartz clock will be used in the GPS. he
speciality of this quartz clock is that it constantly resets. Thus, when
multiple signals from multiple satellites reaches the GPS the clock in
the GPS will gauge its own inaccuracy. Thus only one current value will
be used by the receiver, which causes the incoming signals to contradict
at a single point in space. That time value is held by the atomic
clocks in all satellites. Thus the time value of the receiver clock and
satellite clock will be the same. Though this method is not as accurate
as keeping two atomic clocks you will get almost 99% accuracy. Thus you
get the distance between the satellite and receiver.
Getting the location of both the
satellite and receiver is easier. This is because the satellites travel
at much predictable orbits. So, the GPS has an “ALMANAC” which stores
the data referring to where a particular satellite is positioned at a
particular time. Though the positions change with respect to the pull of
the moon and sun, this is monitored by the Department of defence and is
updated to the GPS receivers.
Differential Global Positioning System (GPS)
Though all these methods are used there
may still be an inaccuracy of (5-10) meters. This can be reduced only by
upgrading the satellites. This is because, the radio signals from these
satellites will be affected by the ionosphere. But, this accuracy
(5-10)meters was obtained only because of Differential GPS.
Differential GPS is essential because of the following factors
- Though the radio signals are known to have the speed of light, it will be reduced as it goes through the ionosphere and the troposphere.
- The radio-signal delay can also depend on your position.
- Bad data from the satellite itself.
Using Differential GPS all these errors
can be corrected. For this a stationary receiver is introduced. This
receivers location is also known and is used to gauge the inaccurate
data. This corrected signal is then provided to all the other
Differential GPS equipped receivers and thus the signal is corrected.
Differential GPS (DGPS) helps correct
these errors. The basic idea is to gauge GPS inaccuracy at a stationary
receiver station with a known location. Since the DGPS hardware at the
station already knows its own position, it can easily calculate its
receiver’s inaccuracy. The station then broadcasts a radio signal to all
DGPS-equipped receivers in the area, providing signal correction
information for that area. In general, access to this correction
information makes DGPS receivers much more accurate than ordinary
receivers.
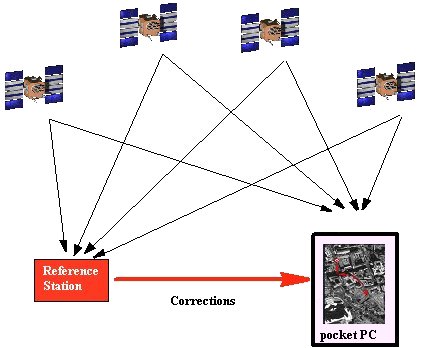
Differential GPS
The raw data output from the GPS is
mostly in latitudes and longitudes. But this is further converted into
map data with the help of map files stored in the memory of GPS.
Advantages of GPS
Apart from our location GPS also helps
us to find the distance we have travelled from one point to your current
position. It can also tell the time you took to reach that distance. It
can also act as a speedometer, and display your average speed.
The size of the circuitory of GPS receivers are very small and so can be taken to any place.
Future of GPS
Though the satellites are capable of
providing the GPS service for all countries, most countries prefer to
stay independent. Most countries are on the verge of launching their own
satellites. India is launching a satellite called IRNSS by 2012. Russia
has its own satellite called GLONASS. Europe will launch their famous
Galileo system by 2013. This is a clear indication that GPS has been
accepted all around the world.

GPS Tracking Circuit Board Assembly
X . II
basic electronic circuits example of a GPS
Of course, the first step in the process is choosing a GPS chip set or module. When considering GPS solutions, there will be a number of factors to consider before the design-in can start.
Can you afford a GPS simulator for testing and production?
Single-channel simulators cost between $10,000 and $15,000. If these costs cannot be accounted for in your product, then an already tested GPS module may be a better choice for your system. You may still need to design 50-Ω RF tracks (more on that later), but the performance of the module comes pre - tested and qualified, albeit at a higher BOM cost.
You may be tempted at this point to “throw an antenna out the window” or use a GPS repeater. Both options will likely result in poor test coverage. A GPS simulator is really your best option for production test.
What are the goals for the GPS antenna, active or passive?
“Active antennas” have a built-in low-noise amplifier (LNA) and will usually connect to your main printed-circuit board (PCB) through a coax cable (either inside your enclosure or outside), while passive antenna designs have no LNA in the antenna element and are commonly mounted directly onto the PCB.
“Passive antenna” designs are more complex and can be susceptible to noise coupling into the antenna ground plane if not correctly isolated from other noise-producing components on the PCB. Testing can also be complicated with passive antenna designs since an RF chamber, re-radiating antenna, and GPS simulator will need to be set up and calibrated for consistent test results.
What type of antenna element do you need? Patch, linear, chip, or something else?
Generally, patch antennas in the 15- to 25-mm size range with at least a 40-mm (on a side) ground plane will give the best performance in portable equipment, but this may be too large for your application. This could force you to look at smaller antenna topologies such as linear or chip antennas.
There’s one thing to note when deciding between chip and patch antennas, though. Patch antennas will provide the best signal performance for their size, as they receive signals on all sides of the patch. Linear GPS antennas (chip or dipole) will generally only receive signals along one of their axes. This results in linear antenna designs being at least half as sensitive (i.e., around –3 dB) compared to patch antennas, and most will probably be around 25% as sensitive as a patch (or about –6 dB).
Some newer chip and folded-F designs show some promise in this area. However, it’s best to evaluate your GPS sensitivity needs with an evaluation kit from your chosen GPS chipset or module manufacturer using various antenna topologies to determine what best fits your design requirements.
If antenna design is not your forte, consider using an active chip or patch antenna module such as those sold by Taoglas Limited. These units provide a tested antenna module while allowing the designer to implement a simple GPS simulator test interface through a U.FL style connector.
Whichever antenna design you choose, you also will need to evaluate the antenna to case spacing for antenna-detuning effects. The manufacturer can tune patch antennas to account for the detuning effects of a plastic case near the patch antenna, but this will likely add cost to your design. Another option is to choose a wider-bandwidth antenna, which usually means a taller antenna.
So you have finally decided to design-in a GPS chipset solution and have chosen an antenna topology. What’s next? First, it may be helpful to have a basic knowledge of GPS signal strength. Signal-wise, the maximum signal strength of a GPS transmission (at ground level) is around –160 dBW (or –130 dBm), which in most receiver designs is going to be about 20 dB below the receiver’s RF noise floor.
Spectrum analyzers and other general RF equipment are not going to detect this signal. In fact, the GPS receiver RF front end will never have an analog signal that can be traced, probed, or captured. The only practical way to detect the presence of the GPS signal is through the correlation process of the GPS receiver itself. As a result, all GPS testing and performance metrics will involve signal data from the GPS receiver, which is an integral part of the test process.
Starting The Design
At this point, you probably have a reference design from your GPS chipset vendor, and you wish to copy its success. The first concept to ingrain in your mental toolbox of design rules is this: the GPS signal is below the noise floor! Repeat this over and over again.
A “quiet” design that passes the electromagnetic compatibility (EMC) tests may not be so quiet as far as the GPS receiver is concerned. The world is fraught with digital noise at this level, so the best design strategy at the start is “isolation.” It won’t guarantee a noise-free design, but it should give you a good start on getting there.
Figure 1 and Figure 2 detail a simplified GPS-receiver schematic and layout including an RF input (including an active antenna supply feed), an LNA, a set of matching components, a surface acoustic wave (SAW) filter, a dc-blocking capacitor, a temperature-controlled crystal oscillator (TCXO), and the GPS RF chip itself, all under the cover of an RF shield can (more on that later).

Usually, in-line resistors inserted in the clock and data paths can control the current flow. The inline resistors minimize the instantaneous current spikes that occur when the signals change state. Another design rule is to remember that the ground connection is a return path for the energy that is being transferred on the signal path. Ground paths should always be present in equal or greater measure to signal paths.
Also, you will need to track in the power supplies to the GPS section on individual traces (not on a power plane). The reason for this rule is that when power and ground planes are placed over each other, they make a plate capacitor with the PCB material acting as the dielectric.
Any noise on the power plane will be coupled directly into the ground plane with noisy results. You may also want to consider using this approach in the rest of your digital design section, as some of the digital noise may find its way up to the GPS RF section through the single-point ground connection.
Strip Line, Via, And Trace Impedance Control
After the component placement is done and your ground planes are defined and isolated, you will need to run your digital, RF, and ground-return traces. For RF signal paths, you will need to set the impedance at 50 Ω. Does this mean you need impedance-controlled PCBs? Not necessarily.
If your PCB manufacturer enables you to set the plane-to-plane spacing (or defines a layer spacing that they will guarantee on a lot-by-lot basis), you can control this yourself. Given an FR4-based PCB material, the necessary trace width can be shown as a function of layer spacing

You will still need a PCB permittivity value to calculate a trace width. Since FR4 generally runs from about 4.3 to 4.7, a value of 4.5 will work in most cases, but you should verify this with your PCB manufacturer. Trace thickness will generally be about 35 µm for 1-oz copper and 17 µm for 0.5-oz copper (typical for inner layers).
When running your RF traces, you will also need to consider the effects that vias will have on the impedance of the trace. At GPS frequencies, each via acts as a small inductor, with the end result being that each via adds around 10 Ω of impedance to your trace. If you use two vias in an RF path (say, one down to an inner layer and then one up to a component pad), you have added 20 Ω of impedance mismatch.
To avoid this, keep all RF traces on the top layer of the PCB (Fig. 2). RF grounds that are not directly tied into the top-layer ground return path will need multiple vias. (Remember, you reduce inductance when adding inductors in parallel.) Power grounds such as decoupling caps can be implemented with single vias, but decoupling components should be as close as possible to the component with a direct-connection, top-layer trace if possible.
Another important note at this point is that it only takes a trace length of about 0.9 in. to make an FR4-based 0.25-wave antenna element (at GPS frequencies), so any long ground traces need to be “shorted” with vias every 0.2 in. or so to keep them from resonating at GPS frequencies. You should also try to place all components end to end and avoid track-stubs wherever possible.
Key Components
The LNA, which is the first stage in the GPS receiver, needs a low-noise power supply to properly function. The easiest way to guarantee low noise is to give the RF its own low-dropout regulator (LDO).
The noise rejection on most commonly available LDOs is in the neighborhood of 50 to 70 dB, and the price is generally under 30 cents in volume, so it’s well worth the cost. You will also need to add some noise isolation (an inductor and cap) between the LNA and the RF supply if it is not already present in your reference design. This protects the LNA from VCO-induced (voltage-controlled oscillator) noise in the rest of the RF.
SAW filters are also necessary in most environments. Follow the matching components guidelines from the reference design, or ask for matching details from the SAW filter provider. Try to preserve the ground connections through the body of the SAW filter (i.e., don’t break the ground underneath the SAW).
A TCXO is a requirement for fast time-to-first-fix (TTFF) and should have an initial tolerance of at least 2.5 ppm. For GPS operation, these oscillators need to be ultra-stable in the 1-Hz time domain—on the order of 1 ppb per second!
Since its short-term stability can be affected by rapid thermal change, the TCXO should be protected from rapidly changing thermal transients. (A shield can be beneficial here; see below.) To meet these requirements, you will need to work with a qualified GPS TCXO provider. A general-purpose TCXO will not do here. Various suppliers are in this market, including Rakon, Kyocera, and ECS.
A shield can, as mentioned above, is necessary for isolating the TCXO from transient thermal conditions. Heat-producing components such as voltage regulators and power transistors should be placed outside of the shield can. Noise-producing components such as switching regulators, high-speed oscillators (other than the GPS TCXO itself), and rapidly switching circuits also should be placed outside of the shield can and away from the RF section in general.
It’s common in VHF and UHF RF shielding to connect all points of the shield can to the PCB’s ground plane. This can be a mistake at GPS frequencies, since the open-air wavelengths of a GPS signal is so much shorter than UHF. Depending on the size of the shield can, if there is current flow across the can, the shield can will be able to resonate near GPS frequencies resulting in interference or de-tuning of the GPS RF.
The simple way to avoid this is to create a shield “ring” that connects to the shield can and then connect that ring to RF ground through an inductor at a single point. The inductor filters any EMI-induced (electromagnetic interference) current flow while the single-point connection prevents current flow across the shield can (and any resulting resonation).
Noise Control (Elsewhere In Your System)
Designing a clean GPS section is not enough if you happen to have noisy components elsewhere on the board. You will also need to look at the edge rates, clocks, and frequencies that are in use by other components. Certain frequencies just won’t work with a GPS receiver on board.
Common interfering frequencies include 4 MHz, usually an IF interferer, and 19.2 MHz. (A multiple falls directly on 1575.42 MHz.) If you have a main-micro running off of a 19.2-MHz oscillator, see if you can change to 24, 25, or 26 MHz, which usually won’t interfere. You can also run your main micro off of the GPS clock if it is a suitable frequency.
Lastly, you will need to look for resistor-cap transmission-line terminations, which puts a low-ohm resistor (<100 Ω) in series with a cap to ground to control impedance and reduce transients (Fig. 3a).

A better approach is to calculate the capacitance of the inputs on the net in question and then insert a resistor in series to set the edge rate (Fig. 3b).
To calculate the edge rate, you can use R-C charging curves. But to simplify things, I’ll present an approximate equation for a 10%/90% switching point:
R = 3t/C
As an example, say we need a 10%/90% switching point with 10-ns rise/fall time for a clock line at 10 MHz (100-ns cycle) and a IC input load capacitance of 10 pF. Substituting values we have:
R = 3t/C
or:
R = 3x10–8/1x10–11
or: 3 kΩ
This may look to be too high a resistance if you’re used to standard RC termination methods, but give it a try. You’ll have much quieter boards as a result! So in summary, follow and review these design rules and you’ll have a good start on implementing a GPS design in your next project.
X . III
What is GPS?

How it works
GPS satellites circle the earth twice a day in a very precise orbit and transmit signal information to earth. GPS receivers take this information and use triangulation to calculate the user's exact location. Essentially, the GPS receiver compares the time a signal was transmitted by a satellite with the time it was received. The time difference tells the GPS receiver how far away the satellite is. Now, with distance measurements from a few more satellites, the receiver can determine the user's position and display it on the unit's electronic map.
How accurate is GPS?
Today's GPS receivers are extremely accurate, thanks to their parallel multi-channel design. Garmin's 12 parallel channel receivers are quick to lock onto satellites when first turned on and they maintain strong locks, even in dense foliage or urban settings with tall buildings. Certain atmospheric factors and other sources of error can affect the accuracy of GPS receivers. Garmin® GPS receivers are accurate to within 15 meters on average.

The GPS satellite system
The 24 satellites that make up the GPS space segment are orbiting the earth about 12,000 miles above us. They are constantly moving, making two complete orbits in less than 24 hours. These satellites are travelling at speeds of roughly 7,000 miles an hour.GPS satellites are powered by solar energy. They have backup batteries onboard to keep them running in the event of a solar eclipse, when there's no solar power. Small rocket boosters on each satellite keep them flying in the correct path.
Here are some other interesting facts about the GPS satellites (also called NAVSTAR, the official U.S. Department of Defense name for GPS):
- The first GPS satellite was launched in 1978.
- A full constellation of 24 satellites was achieved in 1994.
- Each satellite is built to last about 10 years. Replacements are constantly being built and launched into orbit.
- A GPS satellite weighs approximately 2,000 pounds and is about 17 feet across with the solar panels extended.
- Transmitter power is only 50 watts or less.
What's the signal?
GPS satellites transmit two low power radio signals, designated L1 and L2. Civilian GPS uses the L1 frequency of 1575.42 MHz in the UHF band. The signals travel by line of sight, meaning they will pass through clouds, glass and plastic but will not go through most solid objects such as buildings and mountains.A GPS signal contains three different bits of information - a pseudorandom code, ephemeris data and almanac data. The pseudorandom code is simply an I.D. code that identifies which satellite is transmitting information. You can view this number on your Garmin GPS unit's satellite page, as it identifies which satellites it's receiving.
Ephemeris data, which is constantly transmitted by each satellite, contains important information about the status of the satellite (healthy or unhealthy), current date and time. This part of the signal is essential for determining a position.
The almanac data tells the GPS receiver where each GPS satellite should be at any time throughout the day. Each satellite transmits almanac data showing the orbital information for that satellite and for every other satellite in the system.

Sources of GPS signal errors
Factors that can degrade the GPS signal and thus affect accuracy include the following:- Ionosphere and troposphere delays - The satellite signal slows as it passes through the atmosphere. The GPS system uses a built-in model that calculates an average amount of delay to partially correct for this type of error.
- Signal multipath - This occurs when the GPS signal is reflected off objects such as tall buildings or large rock surfaces before it reaches the receiver. This increases the travel time of the signal, thereby causing errors.
- Receiver clock errors - A receiver's built-in clock is not as accurate as the atomic clocks onboard the GPS satellites. Therefore, it may have very slight timing errors.
- Orbital errors - Also known as ephemeris errors, these are inaccuracies of the satellite's reported location.
- Number of satellites visible - The more satellites a GPS receiver can "see," the better the accuracy. Buildings, terrain, electronic interference, or sometimes even dense foliage can block signal reception, causing position errors or possibly no position reading at all. GPS units typically will not work indoors, underwater or underground.
- Satellite geometry/shading - This refers to the relative position of the satellites at any given time. Ideal satellite geometry exists when the satellites are located at wide angles relative to each other. Poor geometry results when the satellites are located in a line or in a tight grouping.
- Intentional degradation of the satellite signal - Selective Availability (SA) is an intentional degradation of the signal once imposed by the U.S. Department of Defense. SA was intended to prevent military adversaries from using the highly accurate GPS signals. The government turned off SA in May 2000, which significantly improved the accuracy of civilian GPS receivers.
X . IIII
SATTELITE TV in digital
( DIGITAL = Direct Gyroscope To Alignment )
1001 ( 2 - an )
When satellite television first hit the market in the early 1990s,
home dishes were expensive metal units that took up a huge chunk of yard
space. In these early years, only the most die-hard TV fans would go
through all the hassle and expense of putting in their own dish.
Satellite TV was a lot harder to get than broadcast and cable TV.
Today, you see compact satellite dishes perched on rooftops all over the United States. Drive through rural
areas beyond the reach of the cable companies, and you'll find dishes
on just about every house. The major satellite TV companies are luring
in more consumers every day with movies, sporting events and news from
around the world and the promise of movie-quality picture and sound.
Satellite TV offers many solutions to broadcast and cable TV problems. Though satellite TV technology is still evolving, it has already become a popular choice for many TV viewers.
In this article, we'll find out how satellite TV works, from TV station to TV set. We'll also learn about the changing landscape of TV viewing and some basic differences that distinguish satellite TV from cable and over-the-air broadcast TV.
Problems with Broadcast TV Conceptually, satellite TV is a lot like broadcast TV. It's a wireless system for delivering television programming directly to a viewer's house. Both broadcast television and satellite stations transmit programming via a radio signal (see How Radio Works for information about radio broadcasting). Broadcast stations use a powerful antenna to transmit radio waves to the surrounding area. Viewers can pick up the signal with a much smaller antenna. The main limitation of broadcast TV is range. The radio signals used to broadcast television shoot out from the broadcast antenna in a straight line. In order to receive these signals, you have to be in the direct line of sight of the antenna. Small obstacles like trees or small buildings aren't a problem; but a big obstacle, such as the Earth, will reflect these radio waves.
If the Earth were perfectly flat, you could pick up broadcast TV thousands of miles from the source. But because the planet is curved, it eventually breaks the signal's line of sight. The other problem with broadcast TV is that the signal is often distorted, even in the viewing area. To get a perfectly clear signal like you find on cable, you have to be pretty close to the broadcast antenna without too many obstacles in the way.
The Satellite TV Solution Satellite TV solves the problems of range and distortion by transmitting broadcast signals from satellites orbiting the Earth. Since satellites are high in the sky, there are a lot more customers in the line of sight. Satellite TV systems transmit and receive radio signals using specialized antennas called satellite dishes.

Satellites are higher in the sky than TV antennas, so they have a much larger line of sight range.
The TV satellites are all in geosynchronous orbit, meaning that they stay in one place in the sky relative to the Earth. Each satellite is launched into space at about 7,000 mph (11,000 kph), reaching approximately 22,200 miles (35,700 km) above the Earth. At this speed and altitude, the satellite will revolve around the planet once every 24 hours -- the same period of time it takes the Earth to make one full rotation. In other words, the satellite keeps pace with our moving planet exactly. This way, you only have to direct the dish at the satellite once, and from then on it picks up the signal without adjustment, at least when everything works right. (See How Satellites Work for more information on satellite orbits.)
At the core, this is all there is to satellite TV. But as we'll see in the next section, there are several important steps between the original programming source and your TV set.
Some satellite owners still seek out this sort of programming on their own, but today, most satellite TV customers get their programming through a direct broadcast satellite (DBS) provider, such as DirecTV or DISH Network. The provider selects programs and broadcasts them to subscribers as a set package. Basically, the provider's goal is to bring dozens or even hundreds of channels to your TV in a form that approximates the competition, cable TV. Unlike earlier programming, the provider's broadcast is completely digital, which means it has much better picture and sound quality. Early satellite television was broadcast in C-band radio -- radio in the 3.7-gigahertz (GHz) to 6.4-GHz frequency range. Digital broadcast satellite transmits programming in the Ku frequency range (11.7 GHz to 14.5 GHz ).
The Components The Components There are five major components involved in a direct to home (DTH) or direct broadcasting (DBS) satellite system: the programming source, the broadcast center, the satellite, the satellite dish and the receiver.
Turnaround channels usually have a distribution center that beams their programming to a geosynchronous satellite. The broadcast center uses large satellite dishes to pick up these analog and digital signals from several sources.
Most local stations don't transmit their programming to satellites, so the provider has to get it another way. If the provider includes local programming in a particular area, it will have a small local facility consisting of a few racks of communications equipment. The equipment receives local signals directly from the broadcaster through fiber-optic cable or an antenna and then transmits them to the central broadcast center.
The broadcast center converts all of this programming into a high-quality, uncompressed digital stream. At this point, the stream contains a vast quantity of data -- about 270 megabits per second (Mbps) for each channel. In order to transmit the signal from there, the broadcast center has to compress it. Otherwise, it would be too big for the satellite to handle. In the next section, we'll find out how the signal is compressed.

A
spy satellite (officially referred to as a reconnaissance satellite or
recon sat) is an Earth observation satellite or communications satellite
deployed for military or intelligence applications. Until the 1970s and
even the 1980s, many reconnaissance satellites that took photographs
would eject canisters of photographic film, which would descend to earth
and be retrieved in mid-air as they floated down on parachutes.
The term "reconnaissance satellite" is preferred, as "spy satellite" often has negative connotations.
In the United States, the most information is available on programs that existed up to 1972. Some information about programs prior to that time is still classified, and a small trickle of information is available on subsequent missions. A few up-to-date reconnaissance satellite images have been declassified on occasion, or leaked, as in the case of KH-11 photographs which were sent to Jane's Defense Weekly in 1985.
History
ARS / SENTRY / SAMOS On 16 March 1955 the Air Force issued General Operational Requirement No. 80, officially establishing a high-level priority for developing an advanced reconnaissance satellite. First designated Advanced Reconnaissance System (ARS), then SENTRY and finally SAMOS the program focused on a system in which a camera equipped satellite would electronically 'scan' images for transmission to ground stations.
Concerns about the length of time required to realize the project's goal led president Eisenhower to approve a Central Intelligence Agency (CIA) satellite reconnaissance system code-named CORONA on 7 February 1958. By the early 1960s all of the various versions of SAMOS had been cancelled leaving CORONA to become the backbone of American satellite reconnaissance for the next decade.
Corona A major reason for the success of CORONA was its relative simplicity. CORONA was designed to take photos then jettison them in a film canister which came back to earth. A specially equipped aircraft would literally snag it out of the air as it descended by parachute.
Mission launches began in 1959 but with sickening regularity they failed mainly due to problems with the launch vehicle including several spectacular explosions. The first success came on the 13th mission when a non-camera carrying CORONA achieved orbit and returned its film canister which was fished out of the ocean.
The first completely successful mission came on 18 August 1960 when a CORONA reached orbit and released a film canister the following day
which was snatched in mid-air. The camera system retroactively
designated KH-1 (KH for KEYHOLE) gave image resolutions on the order of
40ft but with this single mission CORONA provided greater photographic
coverage of the Soviet Union then all of the U-2 spy missions put
together.
The next successful Corona mission on 7 December 1960 carried a more advanced camera system, the KH-2. Improvements continued with the KH-3, KH-4, KH-4A, and finally the KH-4B eventually providing image resolutions of 5-6ft. In addition to CORONA there were two smaller programs ARGON (for mapping) and LANYARD (motivated by a specific target in the Soviet Union) which operated during the years 1962-1964 and 1963 respectively.
Aug. 30, 1961: Corona upgrades to the-KH-3 camera, doubling the level of detail. Feb. 27, 1962: The last launch in the Discoverer series, Discoverer XXXVIII, is a successful debut mission for the new KH 4 camera. The Air Force now imposes a total security blanket on Corona, future launches will be secret, with no effort to maintain a cover story of scientific research.
Corona reaches its prime. From May 1966 through February 1971, 32 launches in a row are either partially or completely successful. May 25, 1972: The final Corona mission is launched, with the final capsule recovered on May 31. During the life of the program, Corona mapped 750 million sguare miles of the Earth's surface, mostly in the Soviet Union and China; the resolution of its cameras improved from initially distinguishing objects on the ground no smaller than 20ft to picking out objects just fiveft across.
Of the 145 total Corona missions, 102 were successful. Altogether, over 860,000 images of the Soviet Union and other parts of the world were delivered. Collection includes 2.1 millionft of film in 39,000 cans.
Argon KH-5 and Lanyard KH-6 ARGON (for mapping); operations: 1962-1964. Of the 11 Argon missions, 6 were successful.
Lanyard (motivated by a specific target in the USSR to meet an emergency requirement for close-up imaging of a suspected Soviet ICBM site near Tallinn.); operations: 1963. Of the 3 Lanyard missions, 1 was successful.
Gambit KH-7, KH-8 Complementing CORONA was a close look or 'spotting' satellite called GAMBIT. Designed to provide high resolution images of small areas rather than broad coverage GAMBIT operated from 1963 into part of 1984. The first camera system for GAMBIT, KH-7 gave a resolution of 18 inches while the second and last camera system, KH-8 had a resolution three times better.

Hexagon
KH-9 'Big Bird'
Designed to succeed CORONA this new generation spy satellite provided
exceptionally detailed images with a resolution of 1-2ft of a much
larger area than CORONA. Between 1971 and 1984 eighteen HEXAGON
satellites were launched. The camera system of HEXAGON was the KH-9.
June 15, 1971: A new-generation spy satellite called "Hexagon" is launched from Vandenberg. It carries a KH-9 camera, capable of exposing more film and covering a wider area on the ground.
KH-10 Dorian MOL (Manned Orbiting Laboratory) An ambitious Air Force project to have a manned reconnaissance platform in outer-space. The MOL was a cylinder with roughly 34 cubic yards of work space. A broad array of tasks were assigned to the MOL but ELINT and reconnaissance duties were certainly intended to be paramount. The project was finally axed in 1969 having consumed over a billion dollars in development.
KH-11 (Kennan / Crystal) In late 1976 a new level of space based surveillance was achieved with the launch of the first KH-11.
KH-12 (KH-11B / Improved Crystal) Similar to the KH-11 but with greatly improved electronics which provide sharper images and a resolution approaching ten centimeters (6 inches) - comparable in quality to the best of the film return satellites. A periscope-like rotating mirror reflects images onto the primary mirror, enabling the KH-12 to take pictures at very high angles of obliquity, imaging objects hundreds of miles away from its flight path. The KH-12 also carries a lot more fuel than the KH-11 (up to 7 tons) giving a longer service life and greater maneuverability.
KH-12 /1 was launched on 28 November 1992 by a Titan-4 from Vandenberg.
KH-12 /2 was launched on 05 December 1995 by a Titan-4 from Vandenberg.
KH-12 /3 was launched on 20 December 1996 by a Titan-4 from Vandenberg.
Indigo / Lacrosse / Vega (Radar Imaging) A space-based imaging radar can see through clouds, and utilization of synthetic aperture radar (SAR) techniques can potentially provide images with a resolution that approaches that of photographic reconnaissance satellites. An project to develop such a satellite was initiated in late 1986 by the CIA. This effort led to the successful test of the Indigo prototype imaging radar satellite in January 1982. Although the decision to proceed with an operational system was controversial, development of the Lacrosse system was approved in 1983.
While Lacrosse is still highly classified and details scarce an imaging resolution of 1m (3.3 ft) is considered feasible for the system.
Lacrosse 1 (1988-106B 19671) was launched on 2 December 1988 by the Space Shuttle. The spacecraft entered an orbit with an inclination of 57 degrees, with an perigee of 680 km and an apogee of 690 km, and has not maneuvered significantly since launch.
Lacrosse 2 (199- A) was launched from Vandenberg AFB CA on a Titan-4 on 08 March 1991
Lacrosse 3 (199- A) was launched from Vandenberg AFB CA on a Titan-4 in the Fall of 1997, replacing Lacrosse 1.
17 August 2000 USA 152 Launch Site: Vandenberg . Launch Vehicle: Titan 4B. Perigee: 689 km. Apogee: 695 km. Inclination: 68.0 deg.
Russian Spy Satellites
Zenit
Zenit-2
Zenit-4
Resurs-F Soviet third-generation Reconnaissance Satellite, an extensive refinement of the earlier ZENIT satellites. The first satellite in this series, the Resurs-Fl, was launched on May 25, 1989. Weighing almost 14,000lb, it deployed two "subsatellites." The main satellite orbited 158 to 170 miles above the earth. It reentered, apparently to return film and other data, on June 17, 1989.

X . IIIIII
Remote sensing is the small- or large-scale acquisition of information
of an object or phenomenon, by the use of either recording or real-time
sensing device(s) that are wireless, or not in physical or intimate
contact with the object (such as by way of aircraft, spacecraft,
satellite, buoy, or ship). In practice, remote sensing is the stand-off
collection through the use of a variety of devices for gathering
information on a given object or area. Thus, Earth observation or
weather satellite collection platforms, ocean and atmospheric observing
weather buoy platforms, the monitoring of a parolee via an ultrasound
identification system, Magnetic Resonance Imaging (MRI), Positron
Emission Tomography (PET), X-radiation (X-RAY) and space probes are all
examples of remote sensing. In modern usage, the term generally refers
to the use of imaging sensor technologies including: instruments found
in aircraft and spacecraft as well as those used in electrophysiology,
and is distinct from other imaging-related fields such as medical
imaging.
Today, you see compact satellite dishes perched on rooftops all over the United States. Drive through rural

Satellite TV offers many solutions to broadcast and cable TV problems. Though satellite TV technology is still evolving, it has already become a popular choice for many TV viewers.
In this article, we'll find out how satellite TV works, from TV station to TV set. We'll also learn about the changing landscape of TV viewing and some basic differences that distinguish satellite TV from cable and over-the-air broadcast TV.
Problems with Broadcast TV Conceptually, satellite TV is a lot like broadcast TV. It's a wireless system for delivering television programming directly to a viewer's house. Both broadcast television and satellite stations transmit programming via a radio signal (see How Radio Works for information about radio broadcasting). Broadcast stations use a powerful antenna to transmit radio waves to the surrounding area. Viewers can pick up the signal with a much smaller antenna. The main limitation of broadcast TV is range. The radio signals used to broadcast television shoot out from the broadcast antenna in a straight line. In order to receive these signals, you have to be in the direct line of sight of the antenna. Small obstacles like trees or small buildings aren't a problem; but a big obstacle, such as the Earth, will reflect these radio waves.
If the Earth were perfectly flat, you could pick up broadcast TV thousands of miles from the source. But because the planet is curved, it eventually breaks the signal's line of sight. The other problem with broadcast TV is that the signal is often distorted, even in the viewing area. To get a perfectly clear signal like you find on cable, you have to be pretty close to the broadcast antenna without too many obstacles in the way.
The Satellite TV Solution Satellite TV solves the problems of range and distortion by transmitting broadcast signals from satellites orbiting the Earth. Since satellites are high in the sky, there are a lot more customers in the line of sight. Satellite TV systems transmit and receive radio signals using specialized antennas called satellite dishes.

Satellites are higher in the sky than TV antennas, so they have a much larger line of sight range.
The TV satellites are all in geosynchronous orbit, meaning that they stay in one place in the sky relative to the Earth. Each satellite is launched into space at about 7,000 mph (11,000 kph), reaching approximately 22,200 miles (35,700 km) above the Earth. At this speed and altitude, the satellite will revolve around the planet once every 24 hours -- the same period of time it takes the Earth to make one full rotation. In other words, the satellite keeps pace with our moving planet exactly. This way, you only have to direct the dish at the satellite once, and from then on it picks up the signal without adjustment, at least when everything works right. (See How Satellites Work for more information on satellite orbits.)
At the core, this is all there is to satellite TV. But as we'll see in the next section, there are several important steps between the original programming source and your TV set.
Satellite TV System
Early satellite TV viewers were explorers of sorts. They used their expensive dishes to discover unique programming that wasn't necessarily intended for mass audiences. The dish and receiving equipment gave viewers the tools to pick up foreign stations, live feeds between different broadcast stations, NASA activities and a lot of other stuff transmitted using satellites.Some satellite owners still seek out this sort of programming on their own, but today, most satellite TV customers get their programming through a direct broadcast satellite (DBS) provider, such as DirecTV or DISH Network. The provider selects programs and broadcasts them to subscribers as a set package. Basically, the provider's goal is to bring dozens or even hundreds of channels to your TV in a form that approximates the competition, cable TV. Unlike earlier programming, the provider's broadcast is completely digital, which means it has much better picture and sound quality. Early satellite television was broadcast in C-band radio -- radio in the 3.7-gigahertz (GHz) to 6.4-GHz frequency range. Digital broadcast satellite transmits programming in the Ku frequency range (11.7 GHz to 14.5 GHz ).
![]() |
The Components The Components There are five major components involved in a direct to home (DTH) or direct broadcasting (DBS) satellite system: the programming source, the broadcast center, the satellite, the satellite dish and the receiver.
- Programming sources are simply the channels that provide programming for broadcast. The provider doesn't create original programming itself; it pays other companies (HBO, for example, or ESPN) for the right to broadcast their content via satellite. In this way, the provider is kind of like a broker between you and the actual programming sources. (Cable TV companies work on the same principle.)
- The broadcast center is the central hub of the system. At the broadcast center, the TV provider receives signals from various programming sources and beams a broadcast signal to satellites in geosynchronous orbit.
- The satellites receive the signals from the broadcast station and rebroadcast them to Earth.
- The viewer's dish picks up the signal from the satellite (or multiple satellites in the same part of the sky) and passes it on to the receiver in the viewer's house.
- The receiver processes the signal and passes it on to a standard TV.
Satellite TV Programming
Satellite TV providers get programming from two major sources: national turnaround channels (such as HBO, ESPN and CNN) and various local channels (the ABC, CBS, Fox, NBC and PBS affiliates in a particular area). Most of the turnaround channels also provide programming for cable TV, and the local channels typically broadcast their programming over the airwaves.Turnaround channels usually have a distribution center that beams their programming to a geosynchronous satellite. The broadcast center uses large satellite dishes to pick up these analog and digital signals from several sources.
Most local stations don't transmit their programming to satellites, so the provider has to get it another way. If the provider includes local programming in a particular area, it will have a small local facility consisting of a few racks of communications equipment. The equipment receives local signals directly from the broadcaster through fiber-optic cable or an antenna and then transmits them to the central broadcast center.
The broadcast center converts all of this programming into a high-quality, uncompressed digital stream. At this point, the stream contains a vast quantity of data -- about 270 megabits per second (Mbps) for each channel. In order to transmit the signal from there, the broadcast center has to compress it. Otherwise, it would be too big for the satellite to handle. In the next section, we'll find out how the signal is compressed.
X . IIIII
Spy Satellites


The term "reconnaissance satellite" is preferred, as "spy satellite" often has negative connotations.
In the United States, the most information is available on programs that existed up to 1972. Some information about programs prior to that time is still classified, and a small trickle of information is available on subsequent missions. A few up-to-date reconnaissance satellite images have been declassified on occasion, or leaked, as in the case of KH-11 photographs which were sent to Jane's Defense Weekly in 1985.
History
ARS / SENTRY / SAMOS On 16 March 1955 the Air Force issued General Operational Requirement No. 80, officially establishing a high-level priority for developing an advanced reconnaissance satellite. First designated Advanced Reconnaissance System (ARS), then SENTRY and finally SAMOS the program focused on a system in which a camera equipped satellite would electronically 'scan' images for transmission to ground stations.
Concerns about the length of time required to realize the project's goal led president Eisenhower to approve a Central Intelligence Agency (CIA) satellite reconnaissance system code-named CORONA on 7 February 1958. By the early 1960s all of the various versions of SAMOS had been cancelled leaving CORONA to become the backbone of American satellite reconnaissance for the next decade.
Corona A major reason for the success of CORONA was its relative simplicity. CORONA was designed to take photos then jettison them in a film canister which came back to earth. A specially equipped aircraft would literally snag it out of the air as it descended by parachute.
Mission launches began in 1959 but with sickening regularity they failed mainly due to problems with the launch vehicle including several spectacular explosions. The first success came on the 13th mission when a non-camera carrying CORONA achieved orbit and returned its film canister which was fished out of the ocean.
The first completely successful mission came on 18 August 1960 when a CORONA reached orbit and released a film canister the following day


The next successful Corona mission on 7 December 1960 carried a more advanced camera system, the KH-2. Improvements continued with the KH-3, KH-4, KH-4A, and finally the KH-4B eventually providing image resolutions of 5-6ft. In addition to CORONA there were two smaller programs ARGON (for mapping) and LANYARD (motivated by a specific target in the Soviet Union) which operated during the years 1962-1964 and 1963 respectively.
Aug. 30, 1961: Corona upgrades to the-KH-3 camera, doubling the level of detail. Feb. 27, 1962: The last launch in the Discoverer series, Discoverer XXXVIII, is a successful debut mission for the new KH 4 camera. The Air Force now imposes a total security blanket on Corona, future launches will be secret, with no effort to maintain a cover story of scientific research.
Corona reaches its prime. From May 1966 through February 1971, 32 launches in a row are either partially or completely successful. May 25, 1972: The final Corona mission is launched, with the final capsule recovered on May 31. During the life of the program, Corona mapped 750 million sguare miles of the Earth's surface, mostly in the Soviet Union and China; the resolution of its cameras improved from initially distinguishing objects on the ground no smaller than 20ft to picking out objects just fiveft across.
Of the 145 total Corona missions, 102 were successful. Altogether, over 860,000 images of the Soviet Union and other parts of the world were delivered. Collection includes 2.1 millionft of film in 39,000 cans.
Argon KH-5 and Lanyard KH-6 ARGON (for mapping); operations: 1962-1964. Of the 11 Argon missions, 6 were successful.
Lanyard (motivated by a specific target in the USSR to meet an emergency requirement for close-up imaging of a suspected Soviet ICBM site near Tallinn.); operations: 1963. Of the 3 Lanyard missions, 1 was successful.
Gambit KH-7, KH-8 Complementing CORONA was a close look or 'spotting' satellite called GAMBIT. Designed to provide high resolution images of small areas rather than broad coverage GAMBIT operated from 1963 into part of 1984. The first camera system for GAMBIT, KH-7 gave a resolution of 18 inches while the second and last camera system, KH-8 had a resolution three times better.


June 15, 1971: A new-generation spy satellite called "Hexagon" is launched from Vandenberg. It carries a KH-9 camera, capable of exposing more film and covering a wider area on the ground.
KH-10 Dorian MOL (Manned Orbiting Laboratory) An ambitious Air Force project to have a manned reconnaissance platform in outer-space. The MOL was a cylinder with roughly 34 cubic yards of work space. A broad array of tasks were assigned to the MOL but ELINT and reconnaissance duties were certainly intended to be paramount. The project was finally axed in 1969 having consumed over a billion dollars in development.
KH-11 (Kennan / Crystal) In late 1976 a new level of space based surveillance was achieved with the launch of the first KH-11.
KH-12 (KH-11B / Improved Crystal) Similar to the KH-11 but with greatly improved electronics which provide sharper images and a resolution approaching ten centimeters (6 inches) - comparable in quality to the best of the film return satellites. A periscope-like rotating mirror reflects images onto the primary mirror, enabling the KH-12 to take pictures at very high angles of obliquity, imaging objects hundreds of miles away from its flight path. The KH-12 also carries a lot more fuel than the KH-11 (up to 7 tons) giving a longer service life and greater maneuverability.
KH-12 /1 was launched on 28 November 1992 by a Titan-4 from Vandenberg.

KH-12 /2 was launched on 05 December 1995 by a Titan-4 from Vandenberg.
KH-12 /3 was launched on 20 December 1996 by a Titan-4 from Vandenberg.
Indigo / Lacrosse / Vega (Radar Imaging) A space-based imaging radar can see through clouds, and utilization of synthetic aperture radar (SAR) techniques can potentially provide images with a resolution that approaches that of photographic reconnaissance satellites. An project to develop such a satellite was initiated in late 1986 by the CIA. This effort led to the successful test of the Indigo prototype imaging radar satellite in January 1982. Although the decision to proceed with an operational system was controversial, development of the Lacrosse system was approved in 1983.
While Lacrosse is still highly classified and details scarce an imaging resolution of 1m (3.3 ft) is considered feasible for the system.
Lacrosse 1 (1988-106B 19671) was launched on 2 December 1988 by the Space Shuttle. The spacecraft entered an orbit with an inclination of 57 degrees, with an perigee of 680 km and an apogee of 690 km, and has not maneuvered significantly since launch.
Lacrosse 2 (199- A) was launched from Vandenberg AFB CA on a Titan-4 on 08 March 1991
Lacrosse 3 (199- A) was launched from Vandenberg AFB CA on a Titan-4 in the Fall of 1997, replacing Lacrosse 1.
17 August 2000 USA 152 Launch Site: Vandenberg . Launch Vehicle: Titan 4B. Perigee: 689 km. Apogee: 695 km. Inclination: 68.0 deg.
Russian Spy Satellites
Zenit
Zenit-2
Zenit-4
Resurs-F Soviet third-generation Reconnaissance Satellite, an extensive refinement of the earlier ZENIT satellites. The first satellite in this series, the Resurs-Fl, was launched on May 25, 1989. Weighing almost 14,000lb, it deployed two "subsatellites." The main satellite orbited 158 to 170 miles above the earth. It reentered, apparently to return film and other data, on June 17, 1989.


X . IIIIII
Satellite Maps
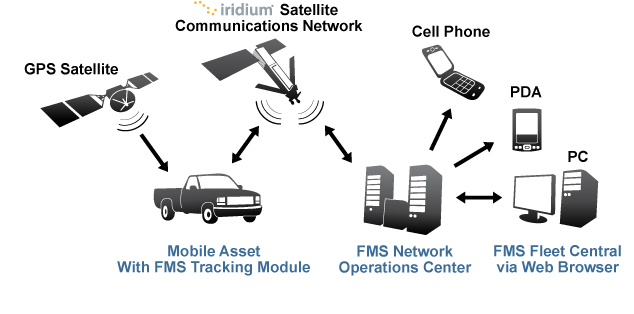




|