Neuralink is an American neurotechnology company founded by Elon Musk and eight others, reported to be developing implantable brain–computer interfaces (BCIs). The company's headquarters are in San Francisco; it was started in 2016 and was first publicly reported in March 2017. The trademark for "Neuralink" was purchased from its previous owners in January 2017.
Wait But Why reported in April 2017 that Musk said the company aims to make devices to treat serious brain diseases in the short-term, with the eventual goal of human enhancement.
In June 2016, Musk discussed a science fiction concept called "neural lace" that is part of the fictional universe in The Culture, a series of novels by Iain M. Banks .
Neuralink, a new company started by Elon Musk (founder of SpaceX , Tesla Motors, and SolarCity), has the goal of interfacing the human brain with machines. Where are we headed?
Why Create Neuralink?
Elon Musk has described some of his ideas publicly. The premise for his latest company, Neuralink, is Musk’s concern that if artificial intelligence (AI) continues to grow at any rate, it will surpass human intelligence by a large amount. He is taking measures to try to increase the probability that the outcome of this would be good rather than bad, but worries that even in a benign scenario humans could end up as the equivalent of pets to an AI, rather than controlling it.Musk believes that one solution to this could involve humans interfacing with machines by adding a digital layer to our brains above the cerebral cortex. He feels that the fundamental limitation of the human brain is the rate at which we input and output information, primarily that our output rate is too slow. By streamlining the transmission of information from brain to computer, Musk believes humans will be able to increase their input/output capabilities, and hopefully avoid being left in the dust by artificial intelligence. The digital layer would not be surgically implanted, but might be injected into the blood stream, and layer itself atop the cortex.
“Neuralink develops high bandwidth and safe brain-machine interfaces.”2 Let’s look at this statement: The high bandwidth refers to the slow input/output rate of humans. The brain interfaces have been around for a while, but they are ever improving.
Where Organisms and Machines Meet
There is a wide range of devices involved in human-machine interfacing. Probably the best known innovator is Kevin Warwick.3 He has made some amazing progress on interfacing himself with machines. Warwick had wonderful success controlling a robotic arm stationed in England while he was in NY, by transmitting his brain waves across the Atlantic Ocean via the internet—see our page The Cyborg Scientist for more on Warwick.Current electronic brain interfaces help prevent seizures in epileptic patients, control tremors in Parkinson patients, improve hearing, improve visual impairment, control prosthetics, or utilize a computer, for example, allowing paraplegics and others to communicate. The basic set-up is shown in the graphic below. Most of the hardware described above is surgically implanted, and often the hardware is eventually rejected or causes damage to nearby tissue, but overall the benefits of these interfaces have outweighed these side-effects.
A brain-computer interface. The person thinks about moving the mouse pointer. The change in electrical activity of the neurons is translated to an electrical impulse that controls the mouse pointer on the computer screen.
The darker regions on the outer layer are the cerebral cortex, which
The two major concerns with any implant are the body rejecting the object and the object damaging surrounding tissue. Most brain implants are rigid and tend to cause damage to the surrounding tissue. In 2016 one scientific group reported5 a way to create a flexible mesh of nanowires whose stiffness is similar to dense neural tissue. The mesh is made of parallel polymer-coated silicon wires, with another set of plain polymer wires perpendicular to these. The mesh curls up and can be injected with a syringe in the region of the cerebral cortex. Once in the vicinity of its destination, the mesh unfurls and lays atop the cortex.
The mesh forms an array of field effect transistors, which detect small changes in electric fields. When the surrounding electric field changes, a current begins to flow from one region of the mesh to another. These transistors are smaller than a neuron, meaning the activity of individual neurons can be mapped. Also, since the mesh is directly in contact with the cerebral cortex, it is able to very accurately detect the firing of neurons deeper in the brain.
The scientists who helped develop the mesh4,5 were able to observe how a mouse’s brain changes as it grows older. This technology mapped the activity of individual neurons over 8 months of the lives of the mice in the study, without rejection by the mouse or damage to tissue.5
Detected electrical changes can be mapped to commands and used to control a prosthetic arm, or a computer. For example, imagine doing an internet search for information about neurons. The thought of the word “neurons” has a distinct electrical pattern, as does the more abstract thought of an internet search. These electrical patterns could be transmitted, via the electronic mesh layer, to a computer. Similarly, you might imagine the information being uploaded by the computer directly to the brain, stimulating the proper neurons involved in making sense of the information. This brings us to input/output and bandwidth.
Bandwidth
What exactly is bandwidth? It turns out there are multiple definitions, but in this situation, bandwidth is how fast information can be transmitted, or the bitrate. If you could simply think about doing an internet search on neurons rather than typing it in, it would already make a big difference in terms of time. That time saving could also go into the transfer rate from computer to our brain if the information could skip our eyes on the way to us.Currently, the human brain outperforms all computers in its calculation time. For example, the fastest computer in the world, Tianhe-2, has a processing speed of 54.902 petaflops—about 55 quadrillion calculations per second6. The human brain is postulated to have 1 exaflop of capacity, or a qunitillion (a thousand quadrillion) calculations per second. Calculations per second is not the issue, yet—the issue is inputting and outputting that information. Think about how fast you type, compared to how fast you create sentences in your head.
Current electronic brain interfaces can’t keep pace with a healthy human’s typing speed, but the technology is rapidly advancing, and already allows people with disabilities to navigate a computer using only their thoughts. One recent study involving brain interfaces focused on typing. In this study, the scientists were interested in a way to increase the output by modifying the digital interface. The subjects were rhesus macaques that used a visual keyboard to transcribe information. The initial interface they used had the monkeys control a cursor with their thoughts, hovering at a symbol on the keyboard to select it. The researchers modified the letter selection to one that utilized brain activity corresponding to thinking about “clicking" on the letter as they viewed it rather than hovering the cursor. This simple modification led to a modest increase in typing rate and bit rate. This study supports the idea that output rates might be increased by improving the digital interface.
As the technology improves, it may one day become possible to move beyond this simple interface and output our sentences just by thinking of them and having a digital interface transmitting those thoughts to a computer.
Manning and Controlling
The interface that allows animals to control a computer with their minds is a one-way device that responds to the input of neural activity, but a different design could allow commands to flow the other direction. According to one Nature article, a group of scientists in Taiwan have mapped out part of the fruit fly’s brain,8 allowing the researchers to control the insect’s head movement or wing movement by using a computer to stimulate a specific set of neural pathways. It took the scientists a full decade to map out 60,000 of the fruit fly’s neurons, less than half its brain, each neuron taking over a gigabyte of data to represent. One neuroscientist estimates that the using the same imaging protocol, it would take over 17 million years to completely map out a human brain. Mapping of the cerebral cortex has already been done, although at a low resolution. High-res mapping of mammalian brains is estimated to take terabytes (trillions of bytes) of data.Dr. Ed Boyden’s team at MIT9 has also made significant progress in recording neural activity. For more information on initial research involving mapping and controlling the brain.
Future Research and Concerns
Elon Musk has a knack for selecting human problems that he can find innovative solutions to: everything from paypal, very drivable electric cars, reusable rockets, and attractive solar roof tiles, to hyperloops, underground transport systems, artificial intelligence, and increasing human capacity via a digital interface. In video of Musk’s discussions he frequently mentions improving the chances of good outcomes for the human race.So research continues on improving artificial intelligence, and making sure it is open access, because Musk believes that power in the hands of many makes a democracy, while in the hands of few makes a system of despotism. And interfacing the brain with technology should keep humans on equal footing with artificial intelligence and those that might control it.
However, concerns of control arise. Hopefully systems will be put in place to take into consideration: Who drives what? Will humans drive machines? Could machines drive humans, or some humans control others through their digital devices? Creating hack-proof hardware as part of the direct human interface would be essential, such as a fundamental boot system that cannot be written over, and can reboot internal digital systems that may become compromised. Otherwise, we may see a day where humans or machines are making other humans turn in circles, flap their arms, or shake their heads, as we have done with fruit flies,mice, and cockroaches.

X . I Neurotechnology.
Neurotechnology is any technology that has a fundamental influence on how people understand the brain and various aspects of consciousness, thought, and higher order activities in the brain. It also includes technologies that are designed to improve and repair brain function and allow researchers and clinicians to visualize the brain.
The field of neurotechnology has been around for nearly half a century but has only reached maturity in the last twenty years. The advent of brain imaging revolutionized the field, allowing researchers to directly monitor the brain's activities during experiments. Neurotechnology has made significant impact on society, though its presence is so commonplace that many do not realize its ubiquity. From pharmaceutical drugs to brain scanning, neurotechnology affects nearly all industrialized people either directly or indirectly, be it from drugs for depression, sleep, ADD, or anti-neurotics to cancer scanning, stroke rehabilitation, and much more.
As the field's depth increases it will potentially allow society to control and harness more of what the brain does and how it influences lifestyles and personalities. Commonplace technologies already attempt to do this; games like BrainAge,[1] and programs like Fast ForWord[2] that aim to improve brain function, are neurotechnologies.
Currently, modern science can image nearly all aspects of the brain as well as control a degree of the function of the brain. It can help control depression, over-activation, sleep deprivation, and many other conditions. Therapeutically it can help improve stroke victims' motor coordination, improve brain function, reduce epileptic episodes (see epilepsy), improve patients with degenerative motor diseases (Parkinson's disease, Huntington's disease, ALS), and can even help alleviate phantom pain perception.[3] Advances in the field promise many new enhancements and rehabilitation methods for patients suffering from neurological problems. The neurotechnology revolution has given rise to the Decade of the Mind initiative, which was started in 2007.[4] It also offers the possibility of revealing the mechanisms by which mind and consciousness emerge from the brain.
Current technologies
Imaging
Magnetoencephalography is a functional neuroimaging technique for mapping brain activity by recording magnetic fields produced by electrical currents occurring naturally in the brain, using very sensitive magnetometers. Arrays of SQUIDs (superconducting quantum interference devices) are the most common magnetometer. Applications of MEG include basic research into perceptual and cognitive brain processes, localizing regions affected by pathology before surgical removal, determining the function of various parts of the brain, and neurofeedback. This can be applied in a clinical setting to find locations of abnormalities as well as in an experimental setting to simply measure brain activity.[5]
Magnetic resonance imaging (MRI) is used for scanning the brain for topological and landmark structure in the brain, but can also be used for imaging activation in the brain.[6] While detail about how MRI works is reserved for the actual MRI article, the uses of MRI are far reaching in the study of neuroscience. It is a cornerstone technology in studying the mind, especially with the advent of functional MRI (fMRI).[7] Functional MRI measures the oxygen levels in the brain upon activation (higher oxygen content = neural activation) and allows researchers to understand what loci are responsible for activation under a given stimulus. This technology is a large improvement to single cell or loci activation by means of exposing the brain and contact stimulation. Functional MRI allows researchers to draw associative relationships between different loci and regions of the brain and provides a large amount of knowledge in establishing new landmarks and loci in the brain.[8]
Computed tomography (CT) is another technology used for scanning the brain. It has been used since the 1970s and is another tool used by neuroscientists to track brain structure and activation.[6] While many of the functions of CT scans are now done using MRI, CT can still be used as the mode by which brain activation and brain injury are detected. Using an X-ray, researchers can detect radioactive markers in the brain that indicate brain activation as a tool to establish relationships in the brain as well as detect many injuries/diseases that can cause lasting damage to the brain such as aneurysms, degeneration, and cancer.
Positron emission tomography (PET) is another imaging technology that aids researchers. Instead of using magnetic resonance or X-rays, PET scans rely on positron emitting markers that are bound to a biologically relevant marker such as glucose.[9] The more activation in the brain the more that region requires nutrients, so higher activation appears more brightly on an image of the brain. PET scans are becoming more frequently used by researchers because PET scans are activated due to metabolism whereas MRI is activated on a more physiological basis (sugar activation versus oxygen activation).
Transcranial magnetic stimulation
Transcranial magnetic stimulation (TMS) is essentially direct magnetic stimulation to the brain. Because electric currents and magnetic fields are intrinsically related, by stimulating the brain with magnetic pulses it is possible to interfere with specific loci in the brain to produce a predictable effect.[10] This field of study is currently receiving a large amount of attention due to the potential benefits that could come out of better understanding this technology.[11] Transcranial magnetic movement of particles in the brain shows promise for drug targeting and delivery as studies have demonstrated this to be noninvasive on brain physiology.[12]Transcranial direct current stimulation
Transcranial direct current stimulation (tDCS) is a form of neurostimulation which uses constant, low current delivered via electrodes placed on the scalp. The mechanisms underlying tDCS effects are still incompletely understood, but recent advances in neurotechnology allowing for in vivo assessment of brain electric activity during tDCS[13] promise to advance understanding of these mechanisms. Research into using tDCS on healthy adults have demonstrated that tDCS can increase cognitive performance on a variety of tasks, depending on the area of the brain being stimulated. tDCS has been used to enhance language and mathematical ability (though one form of tDCS was also found to inhibit math learning),[14] attention span, problem solving, memory,[15] and coordination.Cranial surface measurements
Electroencephalography (EEG) is a method of measuring brainwave activity non-invasively. A number of electrodes are placed around the head and scalp and electrical signals are measured. Typically EEGs are used when dealing with sleep, as there are characteristic wave patterns associated with different stages of sleep.[16] Clinically EEGs are used to study epilepsy as well as stroke and tumor presence in the brain. EEGs are a different method to understand the electrical signaling in the brain during activation.Magnetoencephalography (MEG) is another method of measuring activity in the brain by measuring the magnetic fields that arise from electrical currents in the brain.[17] The benefit to using MEG instead of EEG is that these fields are highly localized and give rise to better understanding of how specific loci react to stimulation or if these regions over-activate (as in epileptic seizures).
Implant technologies
Neurodevices are any devices used to monitor or regulate brain activity. Currently there are a few available for clinical use as a treatment for Parkinson's disease. The most common neurodevices are deep brain stimulators (DBS) that are used to give electrical stimulation to areas stricken by inactivity.[18] Parkinson's disease is known to be caused by an inactivation of the basal ganglia (nuclei) and recently DBS has become the more preferred form of treatment for Parkinson's disease, although current research questions the efficiency of DBS for movement disorders.Neuromodulation is a relatively new field that combines the use of neurodevices and neurochemistry. The basis of this field is that the brain can be regulated using a number of different factors (metabolic, electrical stimulation, physiological) and that all these can be modulated by devices implanted in the neural network. While currently this field is still in the researcher phase, it represents a new type of technological integration in the field of neurotechnology. The brain is a very sensitive organ, so in addition to researching the amazing things that neuromodulation and implanted neural devices can produce, it is important to research ways to create devices that elicit as few negative responses from the body as possible. This can be done by modifying the material surface chemistry of neural implants.
Cell therapy
Researchers have begun looking at uses for stem cells in the brain, which recently have been found in a few loci. A large number of studies are being done to determine if this form of therapy could be used in a large scale. Experiments have successfully used stem cells in the brains of children who suffered from injuries in gestation and elderly people with degenerative diseases in order to induce the brain to produce new cells and to make more connections between neurons.Pharmaceuticals
Pharmaceuticals play a vital role in maintaining stable brain chemistry, and are the most commonly used neurotechnology by the general public and medicine. Drugs like sertraline, methylphenidate, and zolpidem act as chemical modulators in the brain, and they allow for normal activity in many people whose brains cannot act normally under physiological conditions. While pharmaceuticals are usually not mentioned and have their own field, the role of pharmaceuticals is perhaps the most far-reaching and commonplace in modern society (the focus on this article will largely ignore neuropharmaceuticals, for more information, see neuropsychopharmacology). Movement of magnetic particles to targeted brain regions for drug delivery is an emerging field of study and causes no detectable circuit damage.Low field magnetic stimulation
Stimulation with low-intensity magnetic fields is currently under study for depression at Harvard Medical School, and has previously been explored by Bell (et al.),[20] Marino (et al.),[21] and others.How these help study the brain
Magnetic resonance imaging is a vital tool in neurological research in showing activation in the brain as well as providing a comprehensive image of the brain being studied. While MRIs are used clinically for showing brain size, it still has relevance in the study of brains because it can be used to determine extent of injuries or deformation. These can have a significant effect on personality, sense perception, memory, higher order thinking, movement, and spatial understanding. However, current research tends to focus more so on fMRI or real-time functional MRI (rtfMRI).[22] These two methods allow the scientist or the participant, respectively, to view activation in the brain. This is incredibly vital in understanding how a person thinks and how their brain reacts to a person's environment, as well as understanding how the brain works under various stressors or dysfunctions. Real-time functional MRI is a revolutionary tool available to neurologists and neuroscientists because patients can see how their brain reacts to stressors and can perceive visual feedback.[8] CT scans are very similar to MRI in their academic use because they can be used to image the brain upon injury, but they are more limited in perceptual feedback.[6] CTs are generally used in clinical studies far more than in academic studies, and are found far more often in a hospital than a research facility. PET scans are also finding more relevance in academia because they can be used to observe metabolic uptake of neurons, giving researchers a wider perspective about neural activity in the brain for a given condition.[9] Combinations of these methods can provide researchers with knowledge of both physiological and metabolic behaviors of loci in the brain and can be used to explain activation and deactivation of parts of the brain under specific conditions.Transcranial magnetic stimulation is a relatively new method of studying how the brain functions and is used in many research labs focused on behavioral disorders and hallucinations. What makes TMS research so interesting in the neuroscience community is that it can target specific regions of the brain and shut them down or activate temporarily; thereby changing the way the brain behaves. Personality disorders can stem from a variety of external factors, but when the disorder stems from the circuitry of the brain TMS can be used to deactivate the circuitry. This can give rise to a number of responses, ranging from “normality” to something more unexpected, but current research is based on the theory that use of TMS could radically change treatment and perhaps act as a cure for personality disorders and hallucinations.[11] Currently, repetitive transcranial magnetic stimulation (rTMS) is being researched to see if this deactivation effect can be made more permanent in patients suffering from these disorders. Some techniques combine TMS and another scanning method such as EEG to get additional information about brain activity such as cortical response.[23]
Both EEG and MEG are currently being used to study the brain's activity under different conditions. Each uses similar principles but allows researchers to examine individual regions of the brain, allowing isolation and potentially specific classification of active regions. As mentioned above, EEG is very useful in analysis of immobile patients, typically during the sleep cycle. While there are other types of research that utilize EEG,[23] EEG has been fundamental in understanding the resting brain during sleep.[16] There are other potential uses for EEG and MEG such as charting rehabilitation and improvement after trauma as well as testing neural conductivity in specific regions of epileptics or patients with personality disorders.
Neuromodulation can involve numerous technologies combined or used independently to achieve a desired effect in the brain. Gene and cell therapy are becoming more prevalent in research and clinical trials and these technologies could help stunt or even reverse disease progression in the central nervous system. Deep brain stimulation is currently used in many patients with movement disorders and is used to improve the quality of life in patients.[18] While deep brain stimulation is a method to study how the brain functions per se, it provides both surgeons and neurologists important information about how the brain works when certain small regions of the basal ganglia (nuclei) are stimulated by electrical currents.
Future technologies
The future of neurotechnologies lies in how they are fundamentally applied, and not so much on what new versions will be developed. Current technologies give a large amount of insight into the mind and how the brain functions, but basic research is still needed to demonstrate the more applied functions of these technologies. Currently, rtfMRI is being researched as a method for pain therapy. deCharms et al. have shown that there is a significant improvement in the way people perceive pain if they are made aware of how their brain is functioning while in pain. By providing direct and understandable feedback, researchers can help patients with chronic pain decrease their symptoms. This new type of bio/mechanical-feedback is a new development in pain therapy.[8] Functional MRI is also being considered for a number of more applicable uses outside of the clinic. Research has been done on testing the efficiency of mapping the brain in the case when someone lies as a new way to detect lying.[24] Along the same vein, EEG has been considered for use in lie detection as well.[25] TMS is being used in a variety of potential therapies for patients with personality disorders, epilepsy, PTSD, migraine, and other brain-firing disorders, but has been found to have varying clinical success for each condition.[11] The end result of such research would be to develop a method to alter the brain's perception and firing and train patients' brains to rewire permanently under inhibiting conditions (for more information see rTMS).[11] In addition, PET scans have been found to be 93% accurate in detecting Alzheimer's disease nearly 3 years before conventional diagnosis, indicating that PET scanning is becoming more useful in both the laboratory and the clinic.[26]Stem cell technologies are always salient both in the minds of the general public and scientists because of their large potential. Recent advances in stem cell research have allowed researchers to ethically pursue studies in nearly every facet of the body, which includes the brain. Research has shown that while most of the brain does not regenerate and is typically a very difficult environment to foster regeneration,[27] there are portions of the brain with regenerative capabilities (specifically the hippocampus and the olfactory bulbs).[28] Much of the research in central nervous system regeneration is how to overcome this poor regenerative quality of the brain. It is important to note that there are therapies that improve cognition and increase the amount of neural pathways,[2] but this does not mean that there is a proliferation of neural cells in the brain. Rather, it is called a plastic rewiring of the brain (plastic because it indicates malleability) and is considered a vital part of growth. Nevertheless, many problems in patients stem from death of neurons in the brain, and researchers in the field are striving to produce technologies that enable regeneration in patients with stroke, Parkinson's diseases, severe trauma, and Alzheimer's disease, as well as many others. While still in fledgling stages of development, researchers have recently begun making very interesting progress in attempting to treat these diseases. Researchers have recently successfully produced dopaminergic neurons for transplant in patients with Parkinson's diseases with the hopes that they will be able to move again with a more steady supply of dopamine. Many researchers are building scaffolds that could be transplanted into a patient with spinal cord trauma to present an environment that promotes growth of axons (portions of the cell attributed with transmission of electrical signals) so that patients unable to move or feel might be able to do so again.[30] The potentials are wide-ranging, but it is important to note that many of these therapies are still in the laboratory phase and are slowly being adapted in the clinic.[31] Some scientists remain skeptical with the development of the field, and warn that there is a much larger chance that electrical prosthesis will be developed to solve clinical problems such as hearing loss or paralysis before cell therapy is used in a clinic.
Novel drug delivery systems are being researched in order to improve the lives of those who struggle with brain disorders that might not be treated with stem cells, modulation, or rehabilitation. Pharmaceuticals play a very important role in society, and the brain has a very selective barrier that prevents some drugs from going from the blood to the brain. There are some diseases of the brain such as meningitis that require doctors to directly inject medicine into the spinal cord because the drug cannot cross the blood–brain barrier.[33] Research is being conducted to investigate new methods of targeting the brain using the blood supply, as it is much easier to inject into the blood than the spine. New technologies such as nanotechnology are being researched for selective drug delivery, but these technologies have problems as with any other. One of the major setbacks is that when a particle is too large, the patient's liver will take up the particle and degrade it for excretion, but if the particle is too small there will not be enough drug in the particle to take effect. In addition, the size of the capillary pore is important because too large a particle might not fit or even plug up the hole, preventing adequate supply of the drug to the brain.[34] Other research is involved in integrating a protein device between the layers to create a free-flowing gate that is unimpeded by the limitations of the body. Another direction is receptor-mediated transport, where receptors in the brain used to transport nutrients are manipulated to transport drugs across the blood–brain barrier.[35] Some have even suggested that focused ultrasound opens the blood–brain barrier momentarily and allows free passage of chemicals into the brain.[36] Ultimately the goal for drug delivery is to develop a method that maximizes the amount of drug in the loci with as little degraded in the blood stream as possible.
Neuromodulation is a technology currently used for patients with movement disorders, although research is currently being done to apply this technology to other disorders. Recently, a study was done on if DBS could improve depression with positive results, indicating that this technology might have potential as a therapy for multiple disorders in the brain. DBS is limited by its high cost however, and in developing countries the availability of DBS is very limited. A new version of DBS is under investigation and has developed into the novel field, optogenetics. Optogenetics is the combination of deep brain stimulation with fiber optics and gene therapy. Essentially, the fiber optic cables are designed to light up under electrical stimulation, and a protein would be added to a neuron via gene therapy to excite it under light stimuli. So by combining these three independent fields, a surgeon could excite a single and specific neuron in order to help treat a patient with some disorder. Neuromodulation offers a wide degree of therapy for many patients, but due to the nature of the disorders it is currently used to treat its effects are often temporary. Future goals in the field hope to alleviate that problem by increasing the years of effect until DBS can be used for the remainder of the patient's life. Another use for neuromodulation would be in building neuro-interface prosthetic devices that would allow quadriplegics the ability to maneuver a cursor on a screen with their thoughts, thereby increasing their ability to interact with others around them. By understanding the motor cortex and understanding how the brain signals motion, it is possible to emulate this response on a computer screen.
Stem cells[edit]
The ethical debate about use of embryonic stem cells has stirred controversy both in the United States and abroad; although more recently these debates have lessened due to modern advances in creating induced pluripotent stem cells from adult cells. The greatest advantage for use of embryonic stem cells is the fact that they can differentiate (become) nearly any type of cell provided the right conditions and signals. However, recent advances by Shinya Yamanaka et al. have found ways to create pluripotent cells without the use of such controversial cell cultures.[39] Using the patient's own cells and re-differentiating them into the desired cell type bypasses both possible patient rejection of the embryonic stem cells and any ethical concerns associated with using them, while also providing researchers a larger supply of available cells. However, induced pluripotent cells have the potential to form benign (though potentially malignant) tumors, and tend to have poor survivability in vivo (in the living body) on damaged tissue.[40] Much of the ethics concerning use of stem cells has subsided from the embryonic/adult stem cell debate due to its rendered moot, but now societies find themselves debating whether or not this technology can be ethically used. Enhancements of traits, use of animals for tissue scaffolding, and even arguments for moral degeneration have been made with the fears that if this technology reaches its full potential a new paradigm shift will occur in human behavior.Military application
New neurotechnologies have always garnered the appeal of governments, from lie detection technology and virtual reality to rehabilitation and understanding the psyche. Due to the Iraq War and War on Terror, American soldiers coming back from Iraq and Afghanistan are reported to have percentages up to 12% with PTSD. There are many researchers hoping to improve these peoples' conditions by implementing new strategies for recovery. By combining pharmaceuticals and neurotechnologies, some researchers have discovered ways of lowering the "fear" response and theorize that it may be applicable to PTSD. Virtual reality is another technology that has drawn much attention in the military. If improved, it could be possible to train soldiers how to deal with complex situations in times of peace, in order to better prepare and train a modern army.Privacy
Finally, when these technologies are being developed society must understand that these neurotechnologies could reveal the one thing that people can always keep secret: what they are thinking. While there are large amounts of benefits associated with these technologies, it is necessary for scientists, citizens and policy makers alike to consider implications for privacy.[43] This term is important in many ethical circles concerned with the state and goals of progress in the field of neurotechnology (see Neuroethics). Current improvements such as “brain fingerprinting” or lie detection using EEG or fMRI could give rise to a set fixture of loci/emotional relationships in the brain, although these technologies are still years away from full application.[43] It is important to consider how all these neurotechnologies might affect the future of society, and it is suggested that political, scientific, and civil debates are heard about the implementation of these newer technologies that potentially offer a new wealth of once-private information.[43] Some ethicists are also concerned with the use of TMS and fear that the technique could be used to alter patients in ways that are undesired by the patient.X . II Optogenetics
Optogenetics (from Greek optikós, meaning 'seen, visible') is a biological technique which involves the use of light to control cells in living tissue, typically neurons, that have been genetically modified to express light-sensitive ion channels. It is a neuromodulation method employed in neuroscience that uses a combination of techniques from optics and genetics to control and monitor the activities of individual neurons in living tissue—even within freely-moving animals—and to precisely measure these manipulation effects in real-time.[1] The key reagents used in optogenetics are light-sensitive proteins. Neuronal control is achieved using optogenetic actuators like channelrhodopsin, halorhodopsin, and archaerhodopsin, while optical recording of neuronal activities can be made with the help of optogenetic sensors for calcium (GCaMP), vesicular release (synapto-pHluorin), Neurotransmitter (GluSnFRs), or membrane voltage (Arc Lightning, ASAP1).[2] [3] Control or recording is confined to genetically defined neurons and performed in a spatiotemporal-specific manner by light.
The earliest approaches for optogenetic control were invented and applied by Boris Zemelman and Gero Miesenböck,[4][5] at the Sloan-Kettering Cancer Center in New York City, and Dirk Trauner, Richard Kramer and Ehud Isacoff at the University of California, Berkeley; these methods conferred light sensitivity but were never reported to be useful by other laboratories due to the multiple components these approaches required. A distinct single-component approach involving microbial opsin genes introduced in 2005 turned out to be widely applied, as described below. Optogenetics is known for the high spatial and temporal resolution that it provides in altering the activity of specific types of neurons to control a subject's behaviour.
In 2010, optogenetics was chosen as the "Method of the Year" across all fields of science and engineering by the interdisciplinary research journal Nature Methods.[6] At the same time, optogenetics was highlighted in the article on "Breakthroughs of the Decade" in the academic research journal Science.[7] These journals also referenced recent public-access general-interest video Method of the year video and textual SciAm summaries of optogenetics.
Description

Fig 1. Channelrhodopsin-2 (ChR2) induces temporally precise blue light-driven activity in rat prelimbic prefrontal cortical neurons. a) In vitro schematic (left) showing blue light delivery and whole-cell patch-clamp recording of light-evoked activity from a fluorescent CaMKllα::ChR2-EYFP expressing pyramidal neuron (right) in an acute brain slice. b) In vivo schematic (left) showing blue light (473 nm) delivery and single-unit recording. (bottom left) Coronal brain slice showing expression of CaMKllα::ChR2-EYFP in the prelimbic region. Light blue arrow shows tip of the optical fiber; black arrow shows tip of the recording electrode (left). White bar, 100 µm. (bottom right) In vivo light recording of prefrontal cortical neuron in a transduced CaMKllα::ChR2-EYFP rat showing light-evoked spiking to 20 Hz delivery of blue light pulses (right). Inset, representative light-evoked single-unit response.[32]

Fig 2. Halorhodopsin (NpHR) rapidly and reversibly silences spontaneous activity in vivo in rat prelimbic prefrontal cortex. (Top left) Schematic showing in vivo green (532 nm) light delivery and single- unit recording of a spontaneously active CaMKllα::eNpHR3.0- EYFP expressing pyramidal neuron. (Right) Example trace showing that continuous 532 nm illumination inhibits single-unit activity in vivo. Inset, representative single unit event; Green bar, 10 seconds.[32]
A nematode expressing the light-sensitive ion channel Mac. Mac is a proton pump originally isolated in the fungus Leptosphaeria maculans and now expressed in the muscle cells of C. elegans that opens in response to green light and causes hyperpolarizing inhibition. Of note is the extension in body length that the worm undergoes each time it is exposed to green light, which is presumably caused by Mac's muscle-relaxant effects.[33]
Light-Activated Membrane Channels
The hallmark of optogenetics therefore is introduction of fast light-activated channels and enzymes that allow temporally precise manipulation of electrical and biochemical events while maintaining cell-type resolution through the use of specific targeting mechanisms. Among the microbial opsins which can be used to investigate the function of neural systems are the channelrhodopsins (ChR2, ChR1, VChR1, and SFOs) to excite neurons and anion-conducting channelrhodopsins for light-induced inhibition. For silencing, halorhodopsin (NpHR),[37] enhanced halorhodopsins (eNpHR2.0 and eNpHR3.0),[38] archaerhodopsin (Arch), Leptosphaeria maculans fungal opsins (Mac), and enhanced bacteriorhodopsin (eBR) have been employed to inhibit neurons (see Figure 2), including in freely-moving mammals.[39]
Moreover, optogenetic control of well-defined biochemical events within behaving mammals is now also possible. Building on prior work fusing vertebrate opsins to specific G-protein coupled receptors [40] a family of chimeric single-component optogenetic tools was created that allowed researchers to manipulate within behaving mammals the concentration of defined intracellular messengers such as cAMP and IP3 in targeted cells [41] Other biochemical approaches to optogenetics (crucially, with tools that displayed low activity in the dark) followed soon thereafter, when optical control over small GTPases and adenylyl cyclases was achieved in cultured cells using novel strategies from several different laboratories.[42][43][44][45][46] This emerging repertoire of optogenetic probes now allows cell-type-specific and temporally precise control of multiple axes of cellular function within intact animals.[47]
Hardware for Light Application
Another necessary factor is hardware (e.g. integrated fiberoptic and solid-state light sources) to allow specific cell types, even deep within the brain, to be controlled in freely behaving animals. Most commonly, the latter is now achieved using the fiberoptic-coupled diode technology introduced in 2007,[48][49][50] though to avoid use of implanted electrodes, researchers have engineered ways to inscribe a "window" made of zirconia that has been modified to be transparent and implanted in mice skulls, to allow optical waves to penetrate more deeply to stimulate or inhibit individual neurons.[51] To stimulate superficial brain areas such as the cerebral cortex, optical fibers or LEDs can be directly mounted to the skull of the animal. More deeply implanted optical fibers have been used to deliver light to deeper brain areas. Complementary to fiber-tethered approaches, completely wireless techniques have been developed utilizing wirelessly delivered power to headborne LEDs for unhindered study of complex behaviors in freely behaving organisms.
Expression of Optogenetic Actuators
Optogenetics also necessarily includes the development of genetic targeting strategies such as cell-specific promoters or other customized conditionally-active viruses, to deliver the light-sensitive probes to specific populations of neurons in the brain of living animals (e.g. worms, fruit flies, mice, rats, and monkeys). In invertebrates such as worms and fruit flies some amount of retinal isomerase all-trans-retinal (ATR) is supplemented with food. A key advantage of microbial opsins as noted above is that they are fully functional without the addition of exogenous co-factors in vertebrates.
Technique

Three primary components in the application of Optogenetics are as follows (A) Identification or synthesis of a light-sensitive protein (Opsin) such as Channelrhodopsin-2 (ChR2),Halorhodopsin (NpHR), etc... (B) The design of a system to introduce the genetic material containing the opsin into cells for protein expression such as application of Cre-Recombinase or an Adeno-Associated-Virus (C) Application of light emitting instruments.[53]
There is a challenge in introducing the microbial opsin, an optogenetic actuator, into a specific region of the organism in question. A rudimentary approach is to introduce an engineered viral vector that contains the optogenetic actuator gene attached to a recognizable promoter such as CAMKIIα. This allows for some level of specificity as cells that already contain and can translate the given promoter will be infected with the viral vector and hopefully express the optogenetic actuator gene.
Another approach is the creation of transgenic mice where the optogenetic actuator gene is introduced into mice zygotes with a given promoter, most commonly Thy1. Introduction of the optogenetic actuator at an early stage allows for a larger genetic code to be incorporated and as a result, increases the specificity of cells to be infected.
A third and rather novel approach that has been developed is creating transgenic mice with Cre-Recombinase, an enzyme that catalyzes recombination between two lox-P sites. Then by introducing an engineered viral vector containing the optogenetic actuator gene in between two lox-P sites, only the cells containing the Cre-Recombinase will express the microbial opsin. This last technique has allowed for multiple modified optogenetic actuators to be used without the need to create a whole line of transgenic animals every time a new microbial opsin is needed.
After the introduction and expression of the microbial opsin, depending on the type of analysis being performed, application of light can be placed at the terminal ends or the main region where the infected cells are situated. Light stimulation can be performed with a vast array of instruments from light emitting diodes (LEDs) or diode-pumped solid state (DPSS). These light sources are most commonly connected to a computer through a fiber optic cable. Recent advances include the advent of wireless head-mounted devices that also apply LED to targeted areas and as a result give the animal more freedom of mobility to reproduce in vivo results.[54][55]
Issues
Although already a powerful scientific tool, optogenetics, according to Doug Tischer & Orion D. Weiner of the University of California San Francisco, should be regarded as a "first-generation GFP" because of its immense potential for both utilization and optimization.[56] With that being said, the current approach to optogenetics is limited primarily by its versatility. Even within the field of Neuroscience where it is most potent, the technique is less robust on a subcellular level.Selective Expression
One of the main problems of optogenetics is that not all the cells in question may express the microbial opsin gene and this is due to each cell's transcriptional and translational mechanisms, and mutations that may occur. Thus, light activation of a population of cells might not be representable of in vivo occurrences.Building off that limitation, it remains difficult to express opsin in subcellular compartments is far from perfect. Currently, there is an inability to accurately guide the expression of opsin at a subcellular level however there have been developments in membrane trafficking whereby opsin has been successfully integrated into the membrane. Integration of opsin into subcellular compartments such as dendrites, somata or axon terminals will provide a more robust understanding of neuronal circuitry.
Extension of Optogenetic Readouts
An issue with Channelrhodopsin-2 is that its gating properties don't mimic in vivo cation channels of cortical neurons. A solution to this issue with a protein's kinetic property is introduction of variants of Channelrhodopsin-2 with more favorable kinetics.[55][56]Another one of the technique's limitations is that light stimulation produces a synchronous activation of infected cells and this removes any individual cell properties of activation among the population affected. Therefore, it is difficult to understand how the cells in the population affected communicate with one another or how their phasic properties of activation may relate to the circuitry being observed.
Optogenetic studies have been conducted utilizing optogenetic functional magnetic resonance imaging (ofMRI) to elucidate the connectome, a thorough map of the brain’s neural connections. The results, however, are limited by the general properties of fMRI.[57][59] The readouts from this neuroimaging procedure lack the spatial and temporal resolution appropriate for studying the densely packed and rapid-firing neuronal circuits.
Range
The opsin proteins currently in use encompass nearly the entirety of the visual spectrum however the visual spectrum is limited in both penetration and resolution.[57] Successful incorporation of infrared activation will, at a standard irradiance value, increase light penetration and augment resolution through reduction of light scattering.Applications
The field of optogenetics has furthered the fundamental scientific understanding of how specific cell types contribute to the function of biological tissues such as neural circuits in vivo (see references from the scientific literature below). Moreover, on the clinical side, optogenetics-driven research has led to insights into Parkinson's disease[60][61] and other neurological and psychiatric disorders. Indeed, optogenetics papers in 2009 have also provided insight into neural codes relevant to autism, Schizophrenia, drug abuse, anxiety, and depression.Identification of particular neurons and networks
Amygdala
Optogenetic approaches have been used to map neural circuits in the amygdala that contribute to fear conditioning.[65][66][67][68] One such example of a neural circuit is the connection made from the basolateral amydala to the dorsal-medial prefrontal cortex where neuronal oscillations of 4 Hz have been observed in correlation to fear induced freezing behaviors in mice. Transgenic mice were introduced with Channelrhodoposin-2 attached with a Parvalbumin-Cre promoter that selectively infected interneurons located both in the basolateral amygdala and the dorsal-medial prefrontal cortex responsible for the 4 Hz oscillations. The interneurons were optically stimulated generating a freezing behavior and as a result provided evidence that these 4 Hz oscillations may be responsible for the basic fear response produced by the neuronal populations along the dorsal-medial prefrontal cortex and basolateral amygdala.[69]Olfactory Bulb
Optogenetic activation of olfactory sensory neurons was critical for demonstrating timing in odor processing [70] and for mechanism of neuromodulatory mediated olfactory guided behaviors (e.g. aggression, mating) [71] In addition,with the aid of optogenetics, evidence has been reproduced to show that the "afterimage" of odors is concentrated more centrally around the olfactory bulb rather than on the periphery where the olfactory receptor neurons would be located. Transgenic mice infected with channel-rhodopsin Thy1-ChR2, were stimulated with a 473 nm laser transcranially positioned over the dorsal section of the olfactory bulb. Longer photostimulation of mitral cells in the olfactory bulb led to observations of longer lasting neuronal activity in the region after the photostimulation had ceased, meaning the olfactory sensory system is able to undergo long term changes and recognize differences between old and new odors.[72]Nucleus accumbens
Optogenetics, freely moving mammalian behavior, in vivo electrophysiology, and slice physiology have been integrated to probe the cholinergic interneurons of the nucleus accumbens by direct excitation or inhibition. Despite representing less than 1% of the total population of accumbal neurons, these cholinergic cells are able to control the activity of the dopaminergic terminals that innervate medium spiny neurons (MSNs) in the nucleus accumbens.[73] These accumbal MSNs are known to be involved in the neural pathway through which cocaine exerts its effects, because decreasing cocaine-induced changes in the activity of these neurons has been shown to inhibit cocaine conditioning. The few cholinergic neurons present in the nucleus accumbens may prove viable targets for pharmacotherapy in the treatment of cocaine dependence[39]Prefrontal cortex
In vivo and in vitro recordings (by the Cooper laboratory) of individual CAMKII AAV-ChR2 expressing pyramidal neurons within the prefrontal cortex demonstrated high fidelity action potential output with short pulses of blue light at 20 Hz (Figure 1).[32] The same group recorded complete green light-induced silencing of spontaneous activity in the same prefrontal cortical neuronal population expressing an AAV-NpHR vector (Figure 2).Heart
Optogenetics was applied on atrial cardiomyocytes to end spiral wave arrhythmias, found to occur in atrial fibrillation, with light.[74] This method is still in the development stage. A recent study explored the possibilities of optogenetics as a method to correct for arrythmias and resynchronize cardiac pacing. The study introduced Channelrhodopsin-2 into cardiomyocytes in ventricular areas of hearts of transgenic mice and performed in vitro studies of photostimulation on both open-cavity and closed-cavity mice. Photostimulation led to increased activation of cells and thus increased ventricular contractions resulting in increasing heart rates. In addition, this approach has been applied in cardiac resynchronization therapy (CRT) as a new biological pacemaker as a substitute for electrode based-CRT.[75] Lately, optogenetics has been used in the heart to defibrillate ventricular arrhythmias with local epicardial illumination,[76] a generalized whole heart illumination [77] or with customized stimulation patterns based on arrhythmogenic mechanisms in order to lower defibrillation energy.[78]Spiral ganglion
Optogenetic stimulation of the spiral ganglion in deaf mice restored auditory activity.[79] Optogenetic application onto the cochlear region allows for the stimulation or inhibition of the spiral ganglion cells (SGN). In addition, due to the characteristics of the resting potentials of SGN's, different variants of the protein Channelrhodopsin-2 have been employed such as Chronos and CatCh. Chronos and CatCh variants are particularly useful in that they have less time spent in their deactivated states, which allow for more activity with less bursts of blue light emitted. The result being that the LED producing the light would require less energy and the idea of cochlear prosthetics in association with photo-stimulation, would be more feasible.[80]Brainstem
Optogenetic stimulation of a modified red-light excitable channelrhodopsin (ReaChR) expressed in the facial motor nucleus enabled minimally invasive activation of motoneurons effective in driving whisker movements in mice.[81] One novel study employed optogenetics on the Dorsal Ralphe Nucleus to both activate and inhibit dopaminergic release onto the Ventral Tegmental Area. To produce activation transgenic mice were infected with Channelrhodopsin-2 with a TH-Cre promoter and to produce inhibition the hyperpolarizing opsin NpHR was added onto the TH-Cre promoter. Results showed that optically activating dopaminergic neurons led to an increase in social interactions, and their inhibition decreased the need to socialize only after a period of isolation.Precise temporal control of interventions
The currently available optogenetic actuators allow for the accurate temporal control of the required intervention (i.e. inhibition or excitation of the target neurons) with precision routinely going down to the millisecond level. Therefore, experiments can now be devised where the light used for the intervention is triggered by a particular element of behavior (to inhibit the behavior), a particular unconditioned stimulus (to associate something to that stimulus) or a particular oscillatory event in the brain (to inhibit the event). This kind of approach has already been used in several brain regions:Hippocampus
Sharp waves and ripple complexes (SWRs) are distinct high frequency oscillatory events in the hippocampus thought to play a role in memory formation and consolidation. These events can be readily detected by following the oscillatory cycles of the on-line recorded local field potential. In this way the onset of the event can be used as a trigger signal for a light flash that is guided back into the hippocampus to inhibit neurons specifically during the SWRs and also to optogenetically inhibit the oscillation itself [83] These kinds of "closed-loop" experiments are useful to study SWR complexes and their role in memory.Cellular Biology/Cell Signaling Pathways

Optogenetic control of cellular forces and induction of mechanotransduction. Pictured cells receive an hour of imaging concurrent with blue light that pulses every 60 seconds. This is also indicated when the blue point flashes onto the image. The cell relaxes for an hour without light activation and then this cycle repeats again. The square inset magnifies the cell's nucleus.
Photosensitive proteins utilized in various cell signaling pathways
While this extension of optogenetics remains to be further investigated, there are various conceptual methodologies that may prove to immediately robust. There is a considerable body of literature outlining photosensitive proteins that have been utilized in cell signaling pathways.[56] CRY2, LOV, DRONPA and PHYB are photosynthetic proteins involved in inducible protein association whereby activation via light can induce/turn off a signaling cascade via recruitment of a signaling domain to its respective substrate. LOV and PHYB are photosensitive proteins that engage in homodimerization and/or heterodimerization to recruit some DNA-modifying protein, translocate to the site of DNA and alter gene expression levels. CRY2, a protein that inherently clusters when active, has been fused with signaling domains and subsequently photoactivated allowing for clustering-based activation.Proteins LOV and Dronpa have also been adapted to cell signaling manipulation; exposure to light induces conformational changes in the photosensitive protein which can subsequently reveal a previously obscured signaling domain and/or activate a protein that was otherwise allosterically inhibited.Optogenetic Temporal Control of Signals
A different set of signaling cascades respond to stimulus timing duration and dynamics.[95] Adaptive signaling pathways, for instance, adjust in accordance to the current level of the projected stimulus and display activity only when these levels change as opposed to responding to absolute levels of the input.[96] Stimulus dynamics also can trigger activity; treating PC12 cells with epidermal growth factor (inducing a transient profile of ERK activity) leads to cellular proliferation whereas introduction of nerve growth factor (inducing a sustained profile of ERK activity) is associated with a different cellular decision whereby the PC12 cells differentiate into neuron-like cells.[97] This discovery was guided pharmacologically but the finding was replicated utilizing optogenetic inputs instead.[98] This ability to optogenetically control signals for various time durations is being explored to elucidate various cell signaling pathways where there is not a strong enough understanding to utilize either drug/genetic manipulation.
A direct cortical interface with Neuralink technology would consist of creating a layer of artificial intelligence inside the brain.
This layer would continue to grow and learn just as your brain does, as it becomes accustomed to your thoughts and behaviors. The end goal would be humans with higher levels of intelligence and functioning

X . IIII
Neuralink so do concept The Future of Transportation
Seen one on the road yet? Ever thought about getting behind the wheel of one? Depending on the person, the concept of self-driving cars can be scary or exciting. However, no matter your personal stance on automated vehicles, the technology itself is fascinating.
Self-driving cars are on the brink of mainstream reality, with companies such as Google and Tesla currently testing their prototypes. Over the next few years, the way we think about commuting is going to change in front of our eyes.
The Market for Automated Vehicles
Boston Consulting Group has valued the potential market of driverless cars at $77 billion dollars by 2035, while the Brookings Institution believes 1 out of 4 cars on the road will be autonomous by that time.Tesla’s CEO, Elon Musk, has promised a fully autonomous car by 2018. If this comes to fruition, the autonomous car market would have 17 years of traction before 2035. With the pace technology innovation travels at, these predictions seem quite realistic.

Whether or not the U.S. will be the leader in this new market is unclear; with few regulations, China is allowing companies to efficiently test their prototypes in throughout the nation.
China could be the targeted destination for many companies developing automated vehicles because of their lax regulations.
Big Players in the Automated Landscape.
The driverless car market is already a crowded space to enter, with over 33 different tech and auto companies attempting to tap in to this potential market. This group of 33 ranges from traditional automakers to software giants.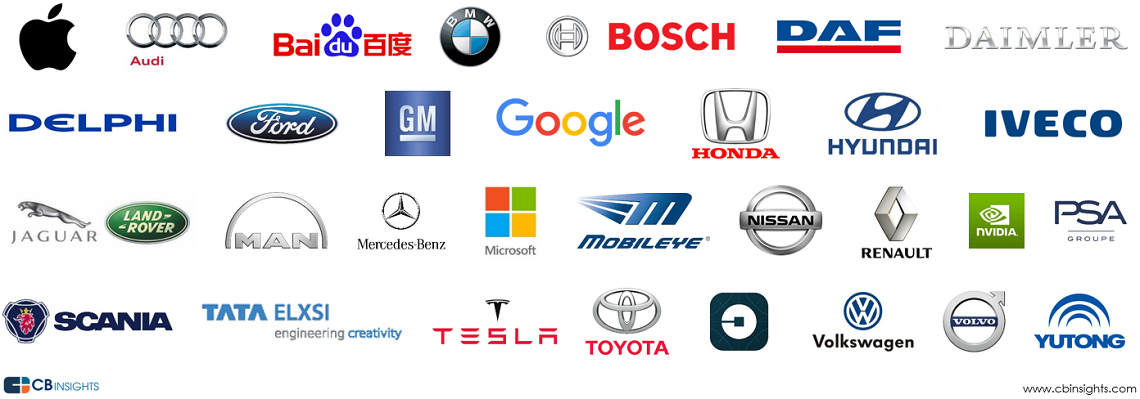
What initially looks like a race between auto and tech companies will likely mold into a cross-collaboration between the two industries over time.
Google’s subsidiary company, Waymo, is one of the industry leaders in self-driving technology. They recently slashed their price by 90% on their LIDAR technology that helps self-driving cars see the world around them. This price cut will help the autonomous market expand, as traditional auto corporations can take advantage of and collaborate with Google’s self-driving technology.
The Problems Behind Automation
Automated vehicles may be the future, but they aren’t all sunshine and daisies just yet. They pose some very serious AI problems that manufacturers are still wrestling with.To overcome skepticism surrounding safety, driverless cars will need to demonstrate far better judgment than humans. Right now, autonomous vehicles are not at that point yet.
Moral Dilemma
There is concern over how AI will make moral decisions for the car. Consider the picture and below scenario.This self-driving vehicle faces two options in an unavoidable accident. Crash into the wall and kill all of its passengers. Or run into the group of pedestrians crossing the street.
Although a rather gruesome picture, this moral dilemma, and many others, will need to be straightened out by the programmers and manufacturers prior to self-driving cars hitting the road.
Weather Conditions
Right now, driverless cars best see the world on a clear and sunny day. They use cameras to detect the basic objects and traffic lights on the road.
To date, there hasn’t been extensive testing in extreme weather conditions, which could pose a problem if a road were covered in snow. There has yet to be a clear direction on how to solve this problem, as the cars will need to adapt to various weather conditions.
Dark Spots
Another AI issue is how cars can detect what New York Times writer Neal E. Boudette classifies as “dark spots.” Recently, automated vehicles are having a tough time distinguishing any dark spot found on the road, whether it is a pothole, puddle, etc.Sound like a simple fix? Resolving this issue requires technology that can analyze these features on the road within a matter of milliseconds. These types of issues shed light on the natural cognitive abilities we have and use while driving, issues that AI will need to become proficient at handling.
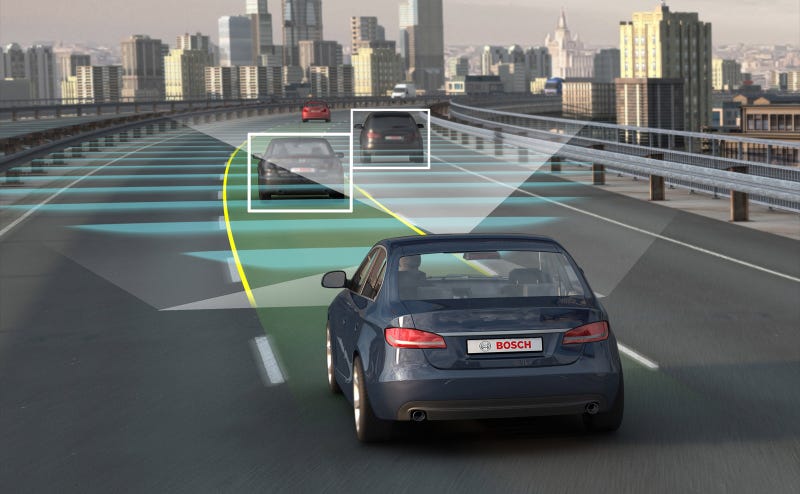
Unpredictable Humans
Furthermore, the problem of unpredictable humans on the road needs to be addressed. These automated vehicles may have no problem following the basic rules of the road under perfect conditions, or when surrounded by other self-driving cars.But what will happen when automated vehicles need to deal with drivers who run red lights or travel well over the speed limit?
A potential solution to this issue is equipping cars with transponders to communicate their position with other vehicles on the road. While this technology is in its early phases, it would allow for automated vehicles to coexist among regular drivers. Going back to the 1 in 4 prediction from earlier, an entirely autonomous driving experience is too far in the future to call.
What Does the Future Hold
Currently, there are many legal and technical issues that still hold back autonomous vehicles from taking over the road. Many of the self-driving companies have set forecasts to release their autonomous vehicles within the next five years.Expect to see more and more prototypes on the road in the coming months, as companies crack down on safety and address the above issues.
A Rosy Picture
While we focused the majority of this article on issues developers are currently handling, we’ll leave you with this mental image:For all the commuters out there, imagine being able to work during your morning drive. Read and respond to emails as your vehicle drives an hour to your office without any guidance. Towards the end of the day, you leave the office an hour early since you can finish everything from your car.
The U.S. has a notoriously long average work week for full-time employees–47 hours. Add-on an hour commute each way, and you’re looking at close to 60 hours a week spent doing work-related activities. Gaining back those hours spent driving to work could be invaluable.
Smart Cities Themselves
Infrastructure changes are the most prominent improvements in the growing trend of smart cities. All cities have infrastructure issues–think traffic–but smart cities are designed to change that. Smart cities offer improved data collection, creating more solutions for city officials. Imagine a world where all city improvements are backed by millions of connected data points from smart infrastructure.An example of smart cities in action can be seen in Columbus, Ohio, the recent winner of the Department of Transportation’s Smart City Challenge. Winning $50 million to invest in smart infrastructure, the city put out a vision for the future, filled with self-driving electric cars and smarter traffic lights.
Utilizing smart city infrastructure, officials hope to transform the transportation service industry in the coming years, with ripple effects sure to affect housing and family lifestyles.
Federal funding is not the only method in which cities will upgrade themselves though. Big tech players are investing too, taking advantage of their resources.
Verizon has invested in a section of Boston, installing fiber networks and other data collection tools. The goal is to collect location-specific data to solve location-specific problems. Cameras, lighting control, and sensors collect information 24/7, such as parking frequency and biking frequency.
Verizon’s investment into this project reveals a major player in the new industry of smart city infrastructure. President-elect Donald Trump has previously stated his support for infrastructure investment, with $1 trillion allocated over 10 years. Verizon better act quickly, as Oracle and IBM are following in their footsteps.
While self-driving cars raise issues of trust, the smart city industry raises privacy issues. With the sensors and integrated networks, corporations could observe us and collect data on our daily patterns.
Even if the public is skeptical about these developments, smart cities’ environmental

Tidak ada komentar:
Posting Komentar