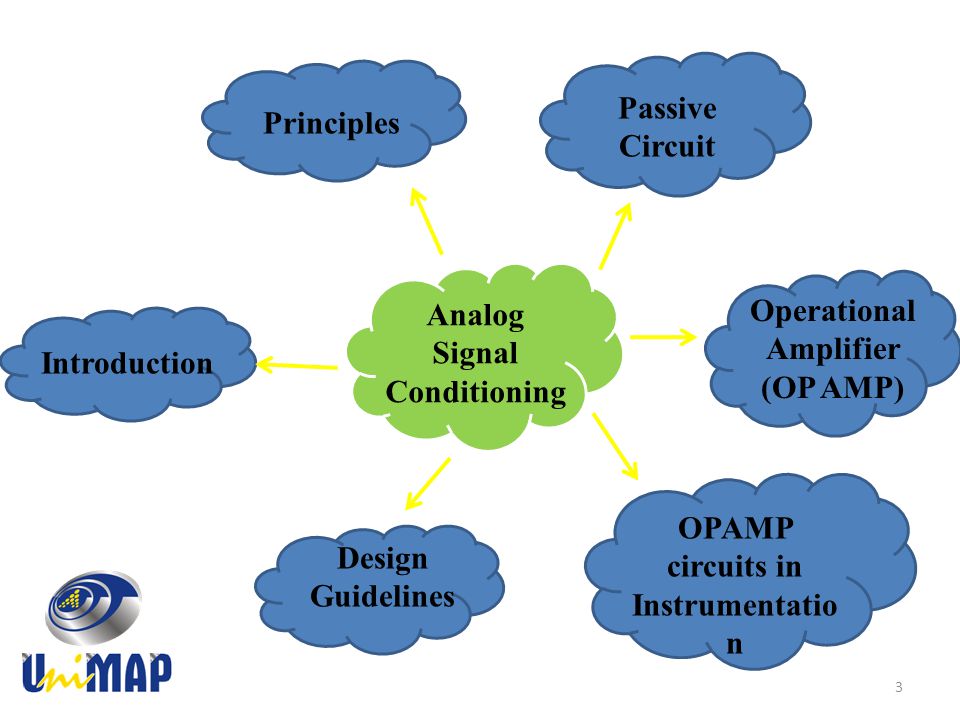
In the field of electronic long-distance transmission with transistor amplification and operational amplifiers, we often encounter in every circuit both in analog circuits and digital circuits, in practice sending and receiving data need to use the above components, although the above components are still applied to the cabling technique but there have been many conditions where the reinforcement system uses wireless networks that conceptualize transistors and operational amplifiers tailored to the transmission media, especially individually or in groups. When we make a signal conditioning technique we are faced with 3 main problems in the MARIA PREFER unit, which is the PREFER reference point where we have to set up a signal conditioning in three modes: open circuit settings, closed loop settings and closed circuit settings control loop, so that the performance of MARIA PREFER ability can have life force and stabilization power for an unlimited period of time and time. but for something like this, material discovery techniques are needed based on the concept of transistors and op-amps as an amplifier, material discovery can be done when we are out of the scope of materials that exist on this earth. once again because so much the Father's Love is in heaven for us.
LOVE AND MARIA PREFER MODE TRANS__OP
( Gen. Mac Tech Zone C O C on MARIA PREFER )
Amplifier, in electronics, device that responds to a small input signal (voltage, current, or power) and delivers a larger output signal that contains the essential waveform features of the input signal. ... The overall amplification of a multistage amplifier is the product of the gains of the individual stages.
A signal conditioner is a device that converts one type of electronic signal into a another type of signal. Its primary use is to convert a signal that may be difficult to read by conventional instrumentation into a more easily read format. In performing this conversion a number of functions may take place.
In electronics, signal conditioning is the manipulation of an analog signal in such a way that it meets the requirements of the next stage for further processing. In an analog-to-digital converter application, signal conditioning includes voltage or current limiting and anti-aliasing filtering.
A signal conditioner is a device that converts one type of electronic signal into a another type of signal. Its primary use is to convert a signal that may be difficult to read by conventional instrumentation into a more easily read format. In performing this conversion a number of functions may take place.
signal conditioning coupler :
1. Filtering of couplings. Signal conditioners protect signal circuits against high-frequency coupling. ... Thus, measurement and control circuits in environments with high-frequency interference are effectively protected.
Most analog signals require some form of preparation before they can be digitized. Signal conditioning is the manipulation of a signal in a way that prepares it for the next stage of processing. Many applications involve environmental or structural measurement, such as temperature and vibration, from sensors.
Signal conditioning is the manipulation of a signal in a way that prepares it for the next stage of processing. Many applications involve environmental or structural measurement, such as temperature and vibration, from sensors.
Signal conditioning plays an important role to achieve signal at a particular level when there are many inputs or outputs between the PLC controller and the outside world and also to isolate it from possible electrical hazards such as high voltages.
Too often taken for granted, signal conditioning is one of the most important components of any data acquisition system. It is the interface between real-world analog signals and the rest of the system. ... A signal conditioner supplies essential circuit elements between the sensor and the data acquisition system.
Signal Conditioning Operations
In previous lectures we have studied various sensors and transducers used in a mechatronics system. Transducers sense physical phenomenon such as rise in temperature and convert the measurand into an electrical signal viz. voltage or current.
signal conditioning work :
The main function of a signal conditioner is to pick up the signal and convert it into a higher level of electrical signal. Signal conversion is often used by industrial applications that use a wide range of sensors to perform measurements.
Signal Amplification. Most cell surface receptors stimulate intracellular target enzymes, which may be either directly linked or indirectly coupled to receptors by G proteins. These intracellular enzymes serve as downstream signaling elements that propagate and amplify the signal initiated by ligand binding.
Cells have proteins called receptors that bind to signaling molecules and initiate a physiological response. ... The names of these receptor classes refer to the mechanism by which the receptors transform external signals into internal ones — via protein action, ion channel opening, or enzyme activation, respectively.
A signal conditioner is a device that converts one type of electronic signal into a another type of signal. Its primary use is to convert a signal that may be difficult to read by conventional instrumentation into a more easily read format. In performing this conversion a number of functions may take place.
Signal isolators provide electrical (galvanic) isolation between the input and output circuits. They couple the signal to the output through a transformer or optical isolator. ... These devices are often used to share, split, boost, protect, step-down, linearize, and digitize process signals.
Active devices :
The active device can be a vacuum tube, discrete solid state component,
such as a single transistor, or part of an integrated circuit, as in an
op-amp). Transistor amplifiers (or solid state amplifiers) are the most
common type of amplifier in use today. A transistor is used as the active element.
Transistor :
Instrumentation Amplifiers (in-amps) are very high gain differential amplifiers which have a high input impedance and a single ended output. Instrumentation amplifiers are mainly used to amplify very small differential signals from strain gauges, thermocouples or current sensing devices in motor control systems.
________________________________________________________________________________
Amplifiers
Electronic amplifiers or "amps" are devices that increase signal power. They are used in everything from radio to computers. Understanding amplifiers is key if you want to be an electrical engineer or simply learn to tweak your audio system. Thousands of engineers have contributed to better amplifier design over the years .
that go into more details on aspects of this topic. 1.) How they work and basics
2.) Power amplifiers
3.) Amplifiers for speakers and music instruments
4.) Transistor amps
5.) Tube amps, how they work
6.) 3 Tube amp model
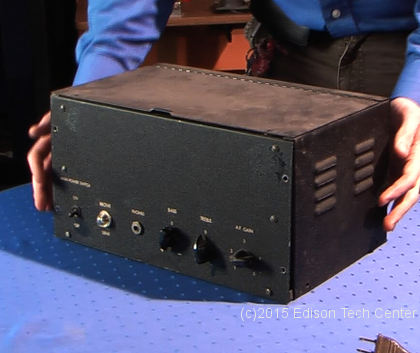
1.) How They Work
In the most basic sense an amplifier takes a weak signal and adds power from a power supply to it to make it larger at the output end.Two basic examples of the need for an amplifiers:
Audio - Thomas Edison and Emile Berliner developed the carbon microphone. DC power passes between two metal plates with carbon in between, one of those plates is the diaphragm that vibrates when soundwaves strike it. This changing distance between the two plates changes the resistance and thus on the output end you have a DC signal that becomes AC as it modulates.
Problem: the output end of a microphone is a weak signal because low DC voltage is needed to make a microphone work. Now we need to take that weak signal and either send it over long distance (like the telephone system) or put it into a loudspeaker. Amplifiers were needed to do this.
Radio - When Alexanderson, Fessenden, Hull and others developed voice radio transmission and radar they needed a way to take the weak radio waves detected by vacuum tubes and amplify the signal so it could power a speaker. Amplifiers (like the triode vacuum tube) were also needed to take weak signals carrying audio and video (television) and turn that signal into either megawatts of signal (for a transmitter), or several watts to power a loudspeaker on the receiver end.
Prerequisites
In order to really understand how amplifiers work and tinker with them yourself you'll need a background in certain areas of electronics. Most engineers begin their life-long passion for the field by tinkering (experimenting with actual devices). If you combine book knowledge with tinkering and safe practices you can master the workings of amplifiers. The most common way to start working in this area is to build your own audio systems, at home or for your car. Amplifiers are very dangerous to work with, the current and voltages involved can kill you so it is important to not work on a powered system and follow safety rules. Even when not plugged in a capacitor can store lots of energy in the system.
Gain - the word gain is used to describe the amplifiers ability to multiply power. To measure gain you need to measure input and output power. Decibels are used to measure gain through equations. Gain is logarithmic, measured by the power of 10.
Oscillators - when an amplifier is connected to a filter and then back to its own input you create a linear oscillator. Oscillators are used in clocks, radio, television, filters, and many other things. They are used to tune circuits, and are important tools for making things operate
2.) Power Amplifiers
In the chain of signal the power amplifier refers to the final amplifier. The power amplifier may boost signal to high levels for use to power an antenna, magnetron (radar), loudspeaker or long distance data transmission wire/fiber.Power amplifiers come in classes to describe how much of the sinusoidal signal is amplified. The amp can be designed to be turned off for half of a cycle, which changes the wave form coming through.
Classes (analogue): A, B, AB, C Classes (digital): D, E, F, G, S, T
Below: Left: tube amplifier for a magnetron in an early microwave oven. The large cylindrical silver and yellow devices are capacitors.
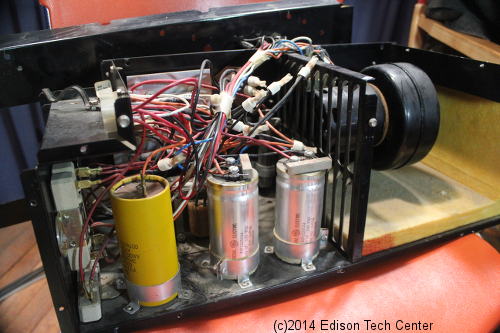
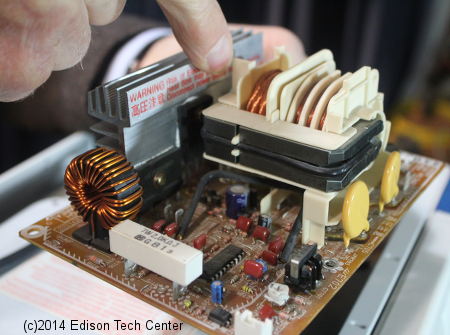
3.) Amplifiers for speakers and music instruments
Amplifiers used to drive speakers take a tiny amount of signal generated by a microphone, radio receiver, television or other device and convert it into a powerful signal enough to drive the strong electromagnets found in speakers.
Audio amplifier circuits are made of:
Capacitors: filter capacitors, coupling capacitors
Resistors
Vacuum tubes or transistors
Rectifiers - converts AC to DC power. Could be silicon diodes or tube rectifiers
Diodes and tube rectifiers
Amplifier circuits to drive electromagnetic speakers were the largest challenge for early audio engineers. Despite the speaker being first theorized in the 1870s it took more than 40 years before we saw the first functional loudspeaker ready for commercial sale. The reason for this is that other technology like vacuum tubes and advanced mathematics for electrical circuits had to be developed to make an audio amplifier that actually produced music and voice as opposed to crude devices that could make a speaker produce an ugly and uncontrolled buzzing noise. Making electronics that could accurately increase audio signal retaining the rich and pure wave forms of the original signal .
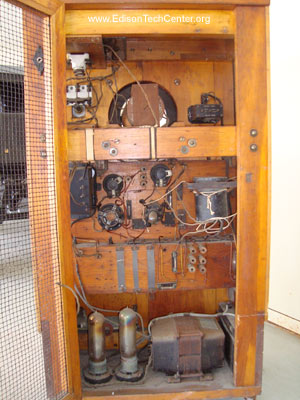
To make an operational amplifier you use multiple transistors along with resistors and capacitors, this way you can amplify a range of frequencies. By applying both negative and positive voltages to the device you can have the amplifier create up to 12 volts(+) with 12 volts(-), this way you have enough power to make a speaker work. There are a 1000 ways to design these circuits but you can start with a few basic models.
Capacitor used before the transistor: transistor amplifiers use a capacitor before the input of the transistor in order to 'center' the DC signal coming from a microphone. Microphones resonate, creating negative and positive DC energy. They also use a 'bias' but for a different reason than transistors. The bias in the microphone energizes the device and puts 0 db up above 0 voltage. The bias in most microphones requires you to supply it with about 2 volts, but it can be different. The capacitor before the transistor brings the 2 volt bias down to actual 0, and therefore removes the DC offset. The transistor needs this to work.
Complications: Creating an amplifier circuit gets complicated due to things like signal noise. We recommend you start building simple amplifiers from kits in order to get the basics down. Than after that you can tweak more powerful and expensive systems.
5.) Using vacuum tubes to amplify
The advent of the triode in 1906 revolutionized telephone and radio. There are many kinds of vacuum tubes used to amplify and we still use some of them today. Tube amps may use tetrodes, triodes and pentode vacuum tubes to do the job of signal amplification.The triode amplifier: This tube has a hot cathode in the center surrounded by a metal grid with the anode surrounding that. The cathode emits electrons, and in the vacuum electrons freely flow through the grid to the anode. By energizing the grid negatively you repel more electrons, this means that less electrons can pass through the grid to get to the anode. If you take weak audio signal (a varying voltage) and apply it to the grid, you'll be letting more power through the grid during positive spikes and less on negative, thus you can greatly amplify the signal.
The bad part about tube amplifiers is that they consume more power and space than transistors. The hot cathode in a tube is made of a tungsten and thorium filament. This filament, just like a lightbulb will burn out after a number of hours and the tube will have to be replaced.
When you attach an amplifier to a speaker the amplifier's behavior will change. Loudspeaker impedance will change as the load changes, and this effects the entire system.
Advantages over transistors: Guitarists will argue that sound from a tube amplifier is better than that from transistor-based amplifiers. Tube amp systems have non-linear clipping and more second-order harmonic distortion, there are plenty of detailed articles out there on this subject. Solid state amplifiers designed for guitarists now use current feedback circuits to increase the output impedance, this gives a similar sound from the speaker that a tube amplifier would.
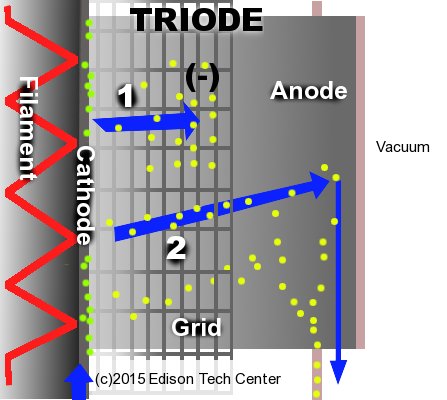
Above: in a triode a filament heats up the cathode, the cathode and the grid are connected to the AC signal.
At (1.) the grid is negatively charged and repels electrons, possibly even blocking them completely from reaching the cathode.
At (2.) the grid is not negatively charged and electrons freely pass through to the curved outer plate which is the cathode.
Note: part (1.) and (2.) are not happening at the same time, they are only shown together for this graphic.
6.) Three Tube Amplifier Example
We will use a simple guitar amplifier with three tubes to demonstrate how the signal is transformed from a weak 0.9 volt signal to a powerful signal enough to power a large speaker diaphragm. Our graphics are a simplified version of the Fender Champ-Amp featured in "Uncle Doug's" videos. See the 38 minute video listed at the bottom here to go into more depth if you need. In our diagrams below we have omitted resistors and most capacitors in order to focus on the action of amplification.
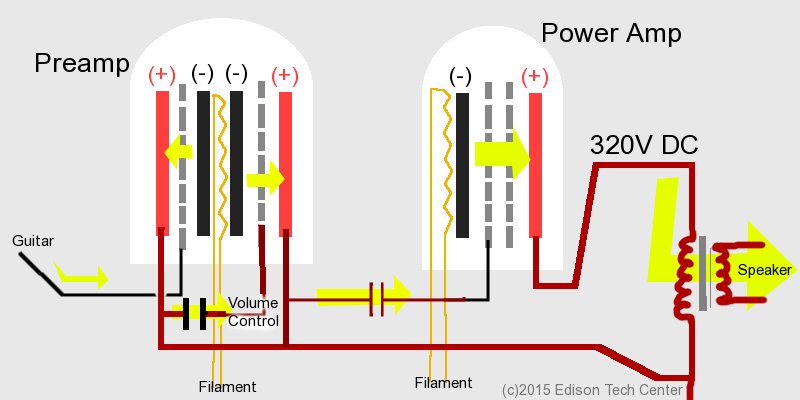
The AC power now follows the DC line from the anode to another grid (12AX7 tube) in the same tube. A capacitor blocks the DC power, only allowing the AC signal through. This signal is now stronger than the original guitar string's signal and this 2nd grid reacts even more strongly, allowing a more extreme AC waveform to pass from cathode to anode. The signal thus has been amplified twice already in this pre-amp tube.
The signal from the pre-amp tube is passed onto the plates of the final tube. The last tube in this chain has a whopping 320 volts DC with an extremely strong + charge. Once again the grid reacts to the AC power and many electrons stream across in the same pattern as the AC waveform. This AC signal passes by a transformer that transforms the power to a voltage that the speaker can use. Normally the 320 volts going through the transformer coil does not effect the speaker's side of the transformer because DC cannot pass through a transformer.
Types of amplifiers:
Operational Amplifiers
Differencial Amplifiers
Isolation Amplifiers
Negative Feedback Amplifiers
Instrumentation Amplifiers
_______________________________________________________________________________
Operational amplifier

An operational amplifier (often op-amp or opamp) is a DC-coupled high-gain electronic voltage amplifier with a differential input and, usually, a single-ended output. In this configuration, an op-amp produces an output potential (relative to circuit ground) that is typically hundreds of thousands of times larger than the potential difference between its input terminals. Operational amplifiers had their origins in analog computers, where they were used to perform mathematical operations in many linear, non-linear, and frequency-dependent circuits.
The popularity of the op-amp as a building block in analog circuits is due to its versatility. By using negative feedback, the characteristics of an op-amp circuit, its gain, input and output impedance, bandwidth etc. are determined by external components and have little dependence on temperature coefficients or engineering tolerance in the op-amp itself.
Op-amps are among the most widely used electronic devices today, being used in a vast array of consumer, industrial, and scientific devices. Many standard IC op-amps cost only a few cents in moderate production volume; however, some integrated or hybrid operational amplifiers with special performance specifications may cost over US$100 in small quantities. Op-amps may be packaged as components or used as elements of more complex integrated circuits.
The op-amp is one type of differential amplifier. Other types of differential amplifier include the fully differential amplifier (similar to the op-amp, but with two outputs), the instrumentation amplifier (usually built from three op-amps), the isolation amplifier (similar to the instrumentation amplifier, but with tolerance to common-mode voltages that would destroy an ordinary op-amp), and negative-feedback amplifier (usually built from one or more op-amps and a resistive feedback network).



A component-level diagram of the common 741 op-amp. Dotted lines outline: current mirrors; differential amplifier; class A gain stage; voltage level shifter; output stage.
Architecture
A small-scale integrated circuit, the 741 op-amp shares with most op-amps an internal structure consisting of three gain stages:- Differential amplifier (outlined dark blue) — provides high differential amplification (gain), with rejection of common-mode signal, low noise, high input impedance, and drives a
- Voltage amplifier (outlined magenta) — provides high voltage gain, a single-pole frequency roll-off, and in turn drives the
- Output amplifier (outlined cyan and green) — provides high current gain (low output impedance), along with output current limiting, and output short-circuit protection.
Op-amps may be classified by their construction:
- discrete (built from individual transistors or tubes/valves)
- IC (fabricated in an Integrated circuit) — most common
- hybrid
- Military, Industrial, or Commercial grade (for example: the LM301 is the commercial grade version of the LM101, the LM201 is the industrial version). This may define operating temperature ranges and other environmental or quality factors.
- Classification by package type may also affect environmental hardiness, as well as manufacturing options; DIP, and other through-hole packages are tending to be replaced by surface-mount devices.
- Classification by internal compensation: op-amps may suffer from high frequency instability in some negative feedback circuits unless a small compensation capacitor modifies the phase and frequency responses. Op-amps with a built-in capacitor are termed "compensated", and allow circuits above some specified closed-loop gain to operate stably with no external capacitor. In particular, op-amps that are stable even with a closed loop gain of 1 are called "unity gain compensated".
- Single, dual and quad versions of many commercial op-amp IC are available, meaning 1, 2 or 4 operational amplifiers are included in the same package.
- Rail-to-rail input (and/or output) op-amps can work with input (and/or output) signals very close to the power supply rails.
- CMOS op-amps (such as the CA3140E) provide extremely high input resistances, higher than JFET-input op-amps, which are normally higher than bipolar-input op-amps.
- other varieties of op-amp include programmable op-amps (simply meaning the quiescent current, bandwidth and so on can be adjusted by an external resistor).
- manufacturers often tabulate their op-amps according to purpose, such as low-noise pre-amplifiers, wide bandwidth amplifiers, and so on.
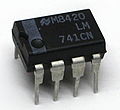
Other applications
- audio- and video-frequency pre-amplifiers and buffers
- differential amplifiers
- differentiators and integrators
- filters
- precision rectifiers
- precision peak detectors
- voltage and current regulators
- analog calculators
- analog-to-digital converters
- digital-to-analog converters
- Voltage clamping
- oscillators and waveform generators
- clipper
- clamper(dc inserter or restorer)
- LOG and ANTILOG amplifiers

Digital Signal Conditioner
Digital Signal Conditioner
Computes Industrial Measurement using Two Sensors

For an industrial measurement application our client needed to use inputs from two sensor inputs to compute a value and produce a proportional analog output. We did so, using an 8-bit PIC® microprocessor from Microchip Inc. The application details are confidential, but here’s the concept.
Each sensor modulates a pulse generating circuit, producing pulses whose width is proportional to its measurement. Similar sensors are used for both measurements: the microprocessor design multiplexes the input between them as shown in the figure. The resultant pulse train’s width varies between “A” and “B”. The microprocessor converts the two pulse widths to digital values.
As part of the project, we developed the equations necessary to perform the required function. The microprocessor performs the calculations. Since this application requires an analog output, the microprocessor converts the result to a duty-cycle-modulated output. The resulting pulse stream is filtered and fed to analog circuitry which produces 4/20mA, 0/5V or 0/10Vdc. For other applications, of course, the output could be purely digital.
Best accuracy requires that the finished product be calibrated together with its mating sensors. We developed a computer program using Visual Basic to do this. During factory calibration the sensors are placed under known conditions. The PIC program measures them and sends their readings to the computer, which calculates and returns calibration constants. After calibration the system is ready for continuous industrial measurement applications.
Tests have shown excellent results which, our client believes, are a step above product currently on the market. The digital signal conditioner was at first manufactured by JH Technology, Inc. as a custom product for their OEM customer but the customer has since bought the rights to the design for their own manufacture.

About the Microprocessor
For this digital instrument design we turned to a Microchip PIC16F series microcontroller because of its many features and also because we were familiar with it from a previous project. It includes peripherals (functions beyond the microprocessor itself) which made the design much easier (and less expensive).
The input pulse is measured by one of the three timers in the PIC®: a second monitors for certain failure modes. A Programmable Switch Mode Controller (PSMC – one of three) produces the output. Computer communication makes use of the serial port (UART). The design also uses two analog comparators, the fixed voltage reference, the D/A converter and one of the three operational amplifiers (op amps) on board.
4 types of cell signaling :
There are four basic categories of chemical signaling found in multicellular organisms: paracrine signaling, autocrine signaling, endocrine signaling, and signaling by direct contact.
________________________________________________________________________________
What is a Signal Conditioner?
Signal Conditioners: Helping to Provide Precise Measurements
A signal conditioner helps to provide precise measurements, which are essential for accurate data acquisition and machine-control. These instruments can perform an additional number of different functions.
Functions of a Signal Conditioner
Signal Conversion
The main function of a signal conditioner is to pick up the signal and convert it into a higher level of electrical signal. Signal conversion is often used by industrial applications that use a wide range of sensors to perform measurements. Due to the different sensors being employed, the signals generated may need to be converted to be usable for the instruments they are connected too. Any sensor signal is capable of being converted to any standard process signal.Linearization
Certain signal conditioners can perform linearization when the signals produced by a sensor do not have a straight-line relationship with the physical measurement. This is the process of interpreting the signal from the software and it is common for thermocouple signals. This method is used to reach higher accuracy because every sensor is not completely linear. The parameters for the linearization are evaluated during the sensor-calibration and mentioned in the calibration protocol of the sensor.Amplifying
The next step is signal amplification and the process of increasing the signal for processing or digitization. There are two ways that signal amplification can be performed; by increasing the resolution of the input signal, or by increasing the signal-to-noise ratio.Signal conditioning uses a range of different amplifiers for different purposes, including instrumentation amplifiers, which are optimised for use with DC signals, and are characterized by high input impedance, high common mode rejection ratio (CMRR), and high gain. Another example of a signal conditioner used for amplification would be an isolation amplifier, which is designed to isolate high DC levels from the device while passing small AC or differential signal.
Filtering
Another important function of a signal conditioner is filtering, and this is where the signal frequency spectrum is filtered to only include the valid data and block any noise. The filters can be made from either passive and active components or digital algorithm. A passive filter only uses capacitors, resistors, and inductors with a maximum gain of one. An active filter uses passive components in addition to active components such as operational amplifiers and transistors. State of the art signal conditioners use digital filters because they are easy to adjust and no hardware is required. A digital filter is a mathematical filter used to manipulate a signal, such as blocking or passing a particular frequency range. They use logic components such as ASICs, FPGAs or in the form of a sequential program with a signal processor.Evaluation and Smart-functions
To provide additional benefits for the user and the process, modern signal conditioners have extra functions for signal evaluation and measurement data preprocessing. This helps to monitor and evaluate warning and alarms directly via an electrical switching output rapidly. Additional Smart-functions like an internal calculated channel can handle mathematical functions, like adding of sensor-signals, up to technological operations like a PID-controller. These functions help to get a fast reacting system and reduce the load from the machine control.Interfaces
Signal converters have to transmit the sensor signals via standard interfaces and protocols to the machine control. These interfaces can be analog or digital. Common analog interfaces are voltage (+/-10V) or current signals (+/-20mA) which are easy to handle but every signal needs a separate wiring. Modern digital interfaces are designed as Ethernet-based bus-interfaces (Profinet, Ethercat, Ethernet/IP) and allow the connection of several components with only one wire. This reduces the wiring and also allows additional information to be transmitted, such as diagnostic information of the components, which is very important for reducing down-times and speed up maintenance.
________________________________________________________________________________
Sensors and Digital Signal Conditioning in Mechatronic Systems
Essential part of a mechatronics system is the measurement system that senses the variations in the physical parameters, such as temperature, pressure, displacement, and so on, and converts it to voltage or current. The control of industrial processes and automated manufacturing systems requests accurate, moreover, linearized sensor measurements, where numerous sensors have nonlinear characteristics. In mechatronic systems, accurate measurement of the dynamic variables plays a vital role for the actuators to function properly. This chapter presents linearization methods and a measurement system in mechatronics consisting of temperature sensors and the signal-conditioning circuits, providing detailed information on design process of an embedded measurement and linearization system. This system uses a 32-bit microcontroller for thermocouple (T/C) cold junction compensation, amplification of low output voltage, then conversion to digital, and linearization of the type K thermocouple’s output by software to output a desired signal. Piecewise and polynomial methods are used in linearization software, and the implemented embedded system for the linearization of a type K T/C is presented as a case study. The obtained results are compared to give an insight to the researchers who work on measurement systems in mechatronics.
Mechatronic systems are composed of mechanical and electrical
components, referred as “smart” systems because of the integration of
sensors, actuators, and control systems. Essential part of a
mechatronics system is the measurement system that senses the variations
in the physical parameters, such as temperature, pressure,
displacement, and so on, and converts it to an electrical quantity, viz.
electric voltage or current. The measurement system consists of mainly
two parts: sensor and signal-conditioning circuit. A device that
displays, records, or controls the measured variable utilizes the output
of the measurement system.
In a measurement system,
sensor detects the change in the physical parameters, but this measured
variable is not usually in the form desired by the rest of the system.
Signal-conditioning part may consist of sensor output amplification,
analog-to-digital conversion (ADC), compensation, frequency-to-voltage
conversion, and so on. The signal-conditioning interface is the part of
the measurement system where the measured signal is converted to an
analog or digital electrical signal that is required by the instrument.
The
control of industrial processes and automated manufacturing systems
requests accurate, moreover, linearized sensor measurements where
numerous sensors have nonlinear characteristics. In mechatronic systems,
accurate measurement of the dynamic variables plays a vital role for
the actuators to function properly. An inaccurate measurement of the
rotational position of a robot arm may result in a misplacement of an
electronic part on a printed circuit board. The nonlinear voltage output
of the temperature sensors embedded in the motor windings results in a
lower temperature reading than the actual temperature, which may cause
shutdown of the motor.
Emerging technologies in
measurement systems have led to integrated signal-conditioning circuits
within the sensors. Spreading the usage of microcontrollers or
microprocessors in sensing technology has increasingly resulted in
utilizing signal-processing functions embedded in the sensors,
consequently accurate and linear signals are available at the outputs.
For this reason, the appropriate selection of linearization technique is
important while the processing time and memory usage of the processor
must be limited for fast response and for maintaining the cost of the
sensor reasonably priced. Linearization and calibration algorithm design
with hardware and/or software is a broad research interest.
Sensors in mechatronics
The
vital part of a mechatronics system is the sensing of physical
parameters—either discrete or continuous. In Electrical Transducer
Nomenclature and Terminology standard, a sensor is defined as “a device
which provides a usable output in response to a specified measurand” . Our focus will be on nonlinear sensors.
In
mechatronic systems, one of the most utilized sensors is the
capacitive-type sensor. Capacitance changes nonlinearly with the
displacement of a diaphragm in a pressure sensor, linear or rotational
motion of an object from the reference, or the displacement of vibration
sensors. Linearization and calibration of this sensor has been an
extensive research area .
The
other utilized sensor is the temperature sensor, which measures the
process temperature, monitor the temperature of the rotor windings, or
in temperature compensation of sensors whose performance is affected by
temperature changes. Temperature is the most measured and controlled
dynamic variable in manufacturing and machine control. There are various
types of these sensors, which use resistance change of metals
(resistance temperature detector (RTD)) and semiconductors (thermistors)
and thermoelectric effect (thermocouples (T/Cs)) due to the change in
temperature. Resistive temperature detectors and thermocouples are the
most preferred ones due to high linearity of the RTDs and wide operating
range of the T/Cs. Although highly nonlinear, thermistors are also used
in temperature sensing due to their high sensitivity and low cost.
Linearization of thermistors has found an extensive interest among
researchers.
Thermistor
is a semiconductor-resistive temperature sensor made from metal oxides
(negative temperature coefficient, NTC) and doped polycrystalline
ceramic containing barium titanate and other compounds (positive
temperature coefficient (PTC)). In NTC thermistors, the resistance
decreases with the increase in temperature. NTC thermistors are widely
used for a narrow range of −50 to 150°C.
Thermocouple is a self-powered sensor where the ends of two
different metals or metal alloys are weld bonded. The bead weld point is
the hot junction, and the other point is the cold junction. A voltage
in the order of millivolts is generated at this cold junction. This
thermoelectric voltage is a function of the difference between the
hot-junction and the cold-junction temperatures and also the composition
of the metals as shown in Figure 2.
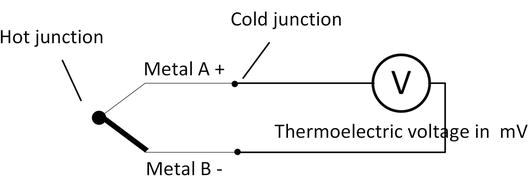
Figure 2.
Thermoelectric voltage of a thermocouple.
This generated voltage by T/C’s cold junction can be
obtained by measuring the junction temperature with a thermistor, or an
IC temperature sensor fixed on an isothermal block. The measured voltage
corresponds to the cold-junction temperature and it is utilized in
computing the hot-junction temperature. The voltage output of the
cold-junction temperature can be fetched from the T/C reference tables
according to IEC 584-1. The difference between this voltage and the
measured voltage is the voltage generated by hot junction. Temperature
value, which corresponds to the computed voltage, can also be fetched
from the T/C temperature/mV output table. This process is the software
compensation.
There are a variety of T/C types ranging
from −270 to 2300°C. They are small in size, thus fast in response, and
low in cost. Unfortunately, they have poor linearity and low
sensitivity. The lead effect is so high that it must be compensated.
Linearization, amplification of the low output, and compensation of the
lead effect of temperature sensors can be carried out by analog circuit
design, , by developed software embedded in a microcontroller or a circuit design where the sampled data stored in an SRAM is transferred to a computer for linearization process Anyway, temperature measurements with T/Cs are challenging, particularly when the temperature measurement is below 0°C.
We
discuss the measurement system in mechatronics consisting of
thermocouples as temperature sensors and the signal-conditioning
circuits, providing detailed information on design process of an
embedded measurement and linearization system. This system uses 32-bit
microcontroller for T/C cold-junction compensation, analog-to-digital
conversion, and T/C temperature sensor output linearization by software.
Methods for linearization
To
overcome the poor linearity of T/Cs, linearization circuits are
developed. Complicated analog electronic circuits are designed to cope
with T/C’s nonlinear and low output voltage problem .
These circuits amplify the low-voltage output to a desired level and
linearize the output to obtain the intended accuracy in the operating
range of the system .
Therefore, in linearization with hardware precise assignation of the
circuit elements is considerably important to achieve the essential
accuracy.
Software linearization techniques are also
preferred among researchers. Wei et al. first amplified the T/C output,
converted it to digital by on-chip analog-to-digital converter of the
microcontroller, and then linearized the output using least-squares
method .
Sarma and Boruah amplified type K T/C output, then converted to digital
by 12-bit ADC, and finally linearized the output with an eight-bit
microcontroller using a piecewise polynomial of ninth degree .
Engin used an eight-bit microcontroller, its on-chip programmable gain
amplifier and 24-bit ADC to amplify and digitize the measured type T T/C
output, and built-in temperature sensor for compensation, finally
linearized the output by first- and second-degree polynomials, and
piecewise linear interpolation methods .
Some researchers utilized a T/C amplifier for amplification and
cold-junction compensation and linearized the output by look-up table
(LUT) embedded in the microcontroller . Wang et al. used B-spline method for linearizing the output of nonlinear sensors .
The sensor linearization process comprises complex mathematical
computations that an eight-bit microcontroller cannot achieve.
Therefore, many researchers had rather realized calibration algorithm on
a computer through I/O interface cards. Danisman et al. initially
amplified the T/C output with an instrumentation amplifier, then used an
ADC for conversion to digital, and transmitted this digitized
measurement to a computer where they applied artificial neural network
(ANN) calibration algorithm by means of a virtual instrument .
Researchers who utilized low-cost microcontrollers for linearizing the
sensor outputs limited the sensor’s input range to a part of the full
scale.
Least-squares regression method
A
set of measured values from a sensor output needs to be fitted to a
curve in order to obtain a mathematical representation of the sensor
output. Linear least-squares regression is considerably the most used
modeling method. This method utilizes linear algebra to determine the
“best-fit” line for a data set by minimizing the sum of the squares of
the vertical residuals of the data points to a modeling curve. The sum
of the squares of the residuals is preferred because this warrants
continuously differentiable residuals at every point contrary to the
absolute error differentiation.
Linear least-squares regression is the main instrument for
process modeling since it is effective in finding a model that best
fits, especially, a small set of data. Although there are sets of data
that are better defined by nonlinear-coefficient functions, numerous
practices in engineering can be described by linear models due to the
fact that these processes are linear in nature or they can be
approximated by a linear model within narrow ranges. In software-based
sensor linearization, it provides minimum code size and consumes the
lowest power.
Sensor linearization
Sensor
outputs are not as linear as we expect. Consequently, linearization by
hardware or software has become one of the challenging parts in
measurement systems.
There are a variety of temperature
sensors in the market. Yet, numerous temperature sensors have nonlinear
characteristics or temperature measuring ranges are quite narrow. While
utilizing these sensors, outputs are compensated, and linearized using
digital circuitry and software, resulting in “smart sensors.” These
smart temperature sensors are thermocouples, thermistors, and resistive
temperature detectors. The temperature sensors have low-voltage outputs,
and nonlinear characteristic as mentioned in Section 2. Amplification
and linearization of the voltage output of these temperature sensors is
essential before utilizing them in industrial applications.
As
the measurement range is the widest, and the linearity is poorer than
most temperature sensors, thermocouples are in our scope of
linearization practice. Due to their robustness to very high/very low
temperatures, and oxidizing environments, they are preferred to other
temperature sensors that melt with high temperature, or corrode with
vapor. We present T/C compensation, and linearization with polynomial
interpolation as a case study.

Sensor linearization procedure.
Hardware
The
suggested temperature measuring system consists of a type K T/C, a
low-voltage micro-power amplifier, OPA333 with very low-offset voltage
(max. 10 µV) and near-zero drift over time, 10 kΩ thermistor for
cold-junction compensation of T/C, a cost-efficient 32-bit
microcontroller, and a serial port driver (Figure ). This system uses an analog hardware- and software-mixed linearization approach.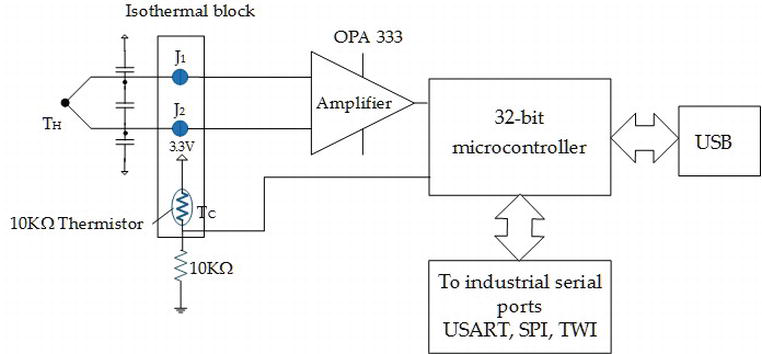
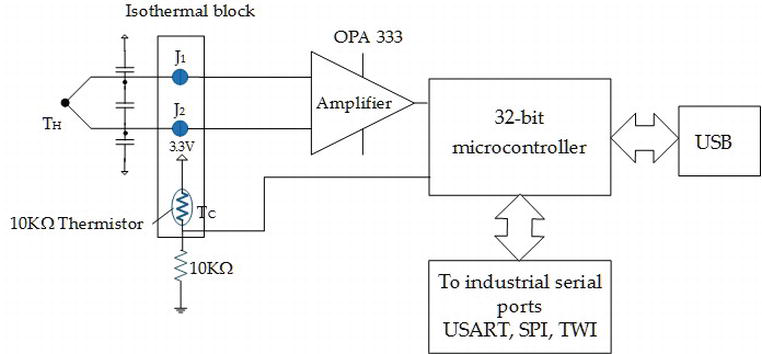
Figure
The low-voltage output of the type K T/C is in the range of
−6.458 to 54.886 mV corresponding to −270 to 1372°C input range, so this
output is amplified by an external amplifier. Then, the amplified
voltage is applied to the built-in ADC input. A low-pass filter is used
for noise suppression across the T/C ends.
We preferred
Arduino Due based on a 32-bit ARM core microcontroller, which has
16-channel 12-bit ADCs; USB, Universal Synchronous/Asynchronous
Receiver/Transmitter (USART), Serial Peripheral Interface (SPI), and I2C
compatible Two-wire Interface (TWI) serial communication ports; 512 KB
of flash and 100 KB of SRAM memory size are sufficient to run the
linearization process by polynomial calculations, or to store the data
for look-up table.
Type | Composition | Temperature range |
---|---|---|
J | Iron vs Cu-Ni alloy | −210 to 1200°C |
K | Ni-Cr alloy vs Ni-Al alloy | −270 to 1372°C |
T | Cu vs Cu-Ni alloy | −270 to 400°C |
E | Ni-Cr alloy vs Cu-Ni alloy | −270 to 1000°C |
Software
Arduino
Due board with ARM Cortex-M3 core can be programmed by its own
integrated development environment (IDE) based on C/C++ programming
language. The flowchart of the algorithm for the temperature measuring
system is given in Figure
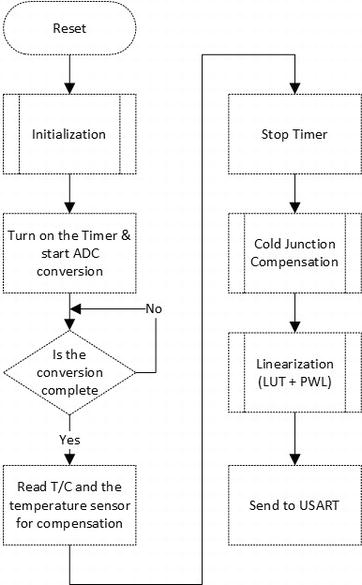
Figure .
Flowchart of the developed software for the temperature measuring system.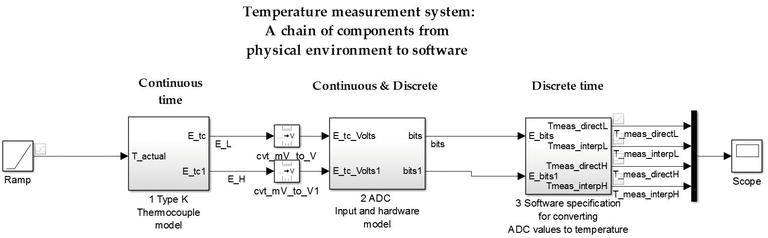
Model for type K T/C measuring system.
Conclusion :
we presented the measurement systems and linearization of sensors as well as the methods used in linearization. We presented the design and simulation process of a type K thermocouple as a case study. The linearization process was implemented with a 32-bit microcontroller. Type K thermocouple was connected to the Arduino Due controller board with an amplifier, and capacitors for the noise-suppression filter. Low-voltage output of the T/C, in the range of −6.458 to 54.886 mV, was amplified by an amplifier, and digitized by an internal 12-bit ADC. As the transmitters are low-power devices, a micro-power amplifier is utilized in the amplification of the output voltage of the T/C.
The
cold-junction compensation of the thermocouple was realized by software
using a thermistor fixed on an isothermal block. The actual type K
thermocouple’s voltage output in the nonlinear range was linearized by
piecewise linear and polynomial interpolation methods, which were used
to compute the temperature values between each breakpoint. Fewer number
of breakpoints resulted in larger errors.
LUTs are used
in a large range of applications including sensor linearization. The
crucial points to consider are the number of LUT entries for better
accuracy and the size of the LUT in design. Fewer number of LUT entries
may cause serious errors in the measurement system, whereas larger
number of entries may consume the memory of the controller.
In
the polynomial approach for linearization, we propose 32-bit
microcontrollers for better accuracy at the expense of cost compared to
the small-embedded systems with limited computing ability and memory
size. The calculation time for high-order polynomial equations may be
too long for low-cost small-embedded systems with limited computational
ability, but for implementation of the exact inverse sensor
characteristic, the 32-bit controller Arduino Due is a reasonable
solution.
Compared to the previous mixed signal works of the authors ,
this case study offers a 32-bit ARM controller with high-computational
ability and memory to run high-order polynomial interpolation method for
linearization as well as higher accuracy in LUT with piecewise
linearization. The ninth degree of polynomial was utilized in T/C
linearization in ,
but the range of linearization was limited to 0–200°C. We proposed a
mixed polynomial linearization that best fits the negative and positive
temperature ranges over the full range.
_______________________________________________________________________________
Signal Conditioners Information
Signal Conditioners Information
The signal conditioning process is also known as a transfer function because the final effect is to convert an input signal (or measurement) into a suitable output signal. For instance, when a temperature sensor measures the temperature of a system or environment, the output of the sensor (temperature) is not suitable to be an input signal to an electrical system. Therefore, the temperature measurement must be converted into an electrical signal.
The following diagram shows the evolution of a signal from the sensor through the central processing unit and to the output or load:
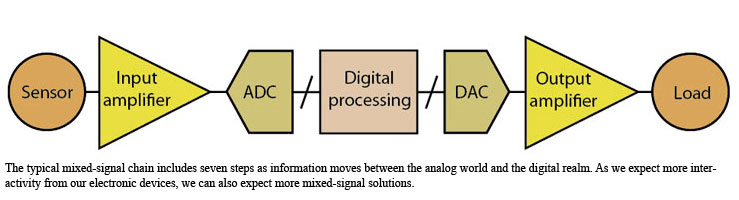
Signal Conditioner Functions
Signal conditioners provide filtering, amplification, converting, and/or other processes required to make sensor outputs suitable for reading by computer boards. They are used primarily for data acquisition, in which sensor signals must be normalized and filtered to levels suitable for analog-to-digital conversion. The digital signal is then available to be analyzed or interpreted by a computerized device.Filtering
Filters can be constructed from either active or passive components. A passive filter uses only resistors, capacitors, and inductors with a maximum gain of one. An active filter uses passive components and active components like operational amplifiers and transistors. They have a higher gain with sharper frequency response curves.The filter architecture can be analog or digital.
Analog (RC) - Analog filters are designed with resistors and capacitors. They are used for analog signals only, and are often used in low-noise requirement applications.
Digital (FIR, IIR) - Digital filters are designed with solid-state components and used for digital signals and quantized signals from a sample-and-hold amplifier. This category includes finite impulse response (FIR) and infinite impulse response (IIR) filters. Digital filtering can approach ideal bandpass characteristics.
Filter Type
The function of the filter is to separate the signal's frequency spectrum into valid data while blocking noise. The standard types of filter responses are low-pass, high-pass, band-pass, and band-reject (or notch filter). Filters are selected based on the frequency of the signal to be analyzed.Low-pass filters block high frequency components; or allow the passage of low frequency signals. A simple passive low-pass filter can be constructed with only a resistor and a capacitor.
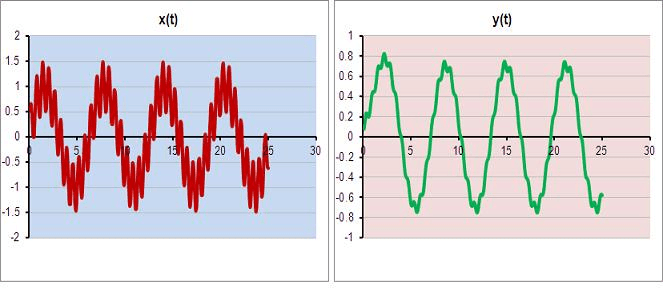
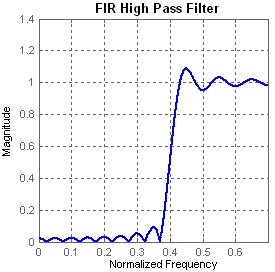
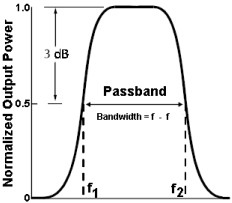
Image Credit: Electronic Engineering Dictionary
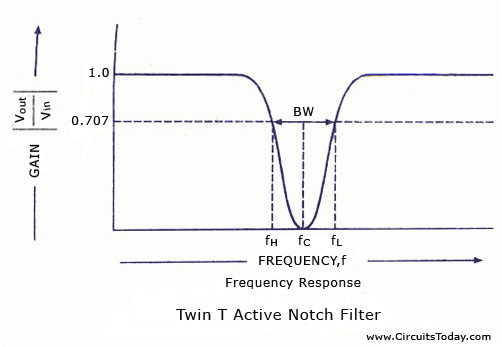
Amplification
Amplification is a process which increases (amplifies) the signal for possessing or digitization. Signal amplifiers often include electronic components that amplify signals without producing significant amounts of thermal noise. In some applications a signal must be amplified or attenuated in order to drive a circuit or a system. There are many types of amplifiers used in signal conditioning including the following:- Voltage followers have a unity gain, so the output signal is a reproduction of the input signal. This type of amplifier is mainly used as an impedance matching device.
- Isolation amplifiers are designed specifically to isolate high DC levels from the data acquisition device while passing the relatively small AC or differential signal. The inputs and outputs are electrically isolated.
- Instrumentation amplifiers are differential amplifiers that have been optimized for use with DC signals. They are characterized by high gain, high common mode rejection ratio (CMRR), and high input impedance.
- Sample-and-hold amplifiers freeze analog voltage instantly. During this process the HOLD command is issued and analog voltage is available for an extended period.
Signal Converting
In many instances it is required to convert a signal from one type to another, in order to accommodate the driving input of circuits. Some important signal converters are:- Current-to-voltage converters scale and convert current signal input to the desired output voltage range.
- Voltage-to-frequency converters accept a voltage signal and convert its analog level to a signal with a corresponding frequency.
- Frequency-to-voltage converters accept a signal and convert its frequency to a corresponding analog voltage level.
- Current loop converters convert an analog or digital signal to a current loop output such as 4-20 mA or 0-20 mA.
- Charge converters convert the charge output from a piezoelectric, capacitive or other charge-producing sensor to a signal such as analog voltage or current.
Digital Conversion
Data acquisition is the digitizing and processing of multiple sensor or signal inputs for the purpose of monitoring, analyzing and/or controlling systems and processes. Analog sensors and signals are first normalized by the use of filters, amplifiers and signal converters. The next wave of the signal chain is the exchange of this signal to a digitized format. The two most important conversion functions in this phase of the process are analog-to-digital conversion and digital-to-analog conversion.Analog-to-Digital ConverterAn analog-to-digital (ADC) converter is a device that accepts, as input, an analog signal and at the output, produces an equivalent digital signal. Most of our sensors and transducers produce analog signals that have to be converted to digital signal in order to be processed by computers or other digital device. There are several types of analog-to-digital (ADC) converters including: direct conversion, successive-approximation, integrating and sigma-delta ADCs.
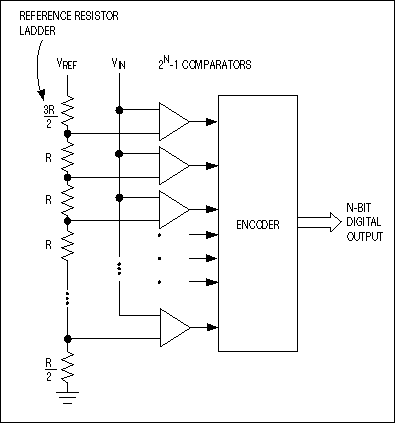
Selection Criteria
After defining the function of the signal conditioner, the form factor, device specifications, signal inputs, sensor inputs, excitation, outputs and user interface are important parameters to consider when searching for signal conditioners.Form Factor
Common form factors for signal conditioners include circuit board, panel or chassis mount, modular bay or slot system, rack mount, DIN rail, and stand-alone.- Printed circuit boards(PCBs) attach to enclosures or plug into computer backplanes.
- Panel or chassis mounts are used to install the device in cabinets, enclosures, or panels with bolts.
- Modular style units include stackable units that dock in bays, slots, or boxes.
- Rack units that fit inside a standard 19” telecommunications rack.
- Devices can be designed for mount on a Standard Deutsches Institute for Normung (DIN) rail, which is a German standard.
- Benchtop or freestanding devices often feature full casings or cabinets and integral interfaces.
Device Specifications
Device specifications that are important to consider when searching for signal conditioning products include analog input channels, digital I/O channels, and accuracy.Analog signals are a wave signal which means that the value changes steadily over time and can have any value in a range. Signal converters with analog inputs can have multiple channels. Channels are either single-end or differential.
Single-end inputs have only one low wire shared by all inputs. For example a board could have 2 single-end inputs; there will be two input lines and one ground line. Single-end inputs are less expensive and allow for twice the number of inputs in the same size wiring connector since they only require one analog input and one ground input which is shared by all the inputs. They save space and are easier to install. When single-ended outputs are available, suppliers often specify the maximum number of analog channel outputs as twice the number of differential outputs.
Differential channels have two inputs. The voltage is the signal processed between the two inputs. The board will have one signal and one ground pin for each input to allow for measurement voltage difference between two signals tied to the same ground. Differential channels provide excellent common-mode noise rejection. This type of input should be used when EMI, RIF or noise is present.
Digital signals do not have 'in between' values. They are an on or off signal producing a square wave. Digital signals break down the information into binary code, which is a series of 1sand 0s. The data receiver reassembles the code into useful information. Digital signal allows users to send more information in a smaller space.
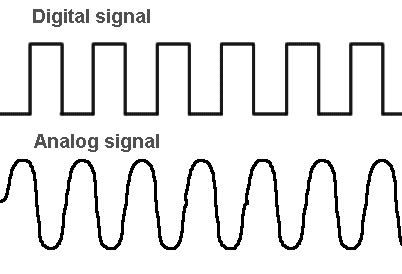
Image credit: Being Ministry
Static accuracy is the combined effects of Linearity, Hysteresis, and Repeatability. It is expressed as +/- percentage of full scale output. The static error band is a good measure of the accuracy that can be expected at constant temperature.
- Linearity is the deviation of a calibration curve from a specified straight line. One way to measure linearity is to use the least squares method, which gives a best fit straight line. The best straight line (BSL) is a line between two parallel lines that enclose all output vs. pressure values on the calibration curve.
- Repeatability is the ability of a transducer to reproduce output readings when the same pressure is applied to the transducer repeatedly, under the same conditions and in the same direction.
- Hysteresis is the maximum difference in output at any pressure within the specified range, when the value is first approached with increasing and then with decreasing pressure. Temperatures hysteresis is the sensor's ability to give the same output at a given temperature before and after a temperature cycle.
Image credit: Showa Measuring Instruments Co. LTD
Signal Inputs
The input signal can have a variety of specifications as it enters the converter. The type of converter selected depends largely on the type of input signal from the system and the desired output signal. The input signal could have properties such as:- DC type voltage and/or current
- AC type voltage and/or current
- Frequency waveforms for varying frequency, pulse or specialized waveforms.
- Chargewhich comes from a piezoelectric device and usually requires conditioning.
Sensor Inputs
Sensor inputs can be accelerometer, thermocouple, thermistor, RTD, strain gauge or bridge, and LVDT or RVDT. Specialized inputs include encoder, counter or tachometer, timer or clock, relay or switch, and other specialized inputs.Excitation
Sensors can be classified as either active or passive devices. Passive devices like thermocouples can generate a signal without a power supply. Active sensors need a power supply in order to control the flow of electrons and make a measurement. In some cases active sensors are powered by the signal conditioner. The output from the signal conditioner that powers the device is referred to as an excitation source. Signal excitation can either be a voltage or current output. The following schematic showcases how an excitation voltage powers a Wheatstone bridge: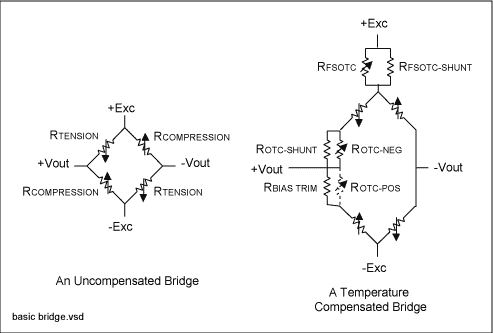
Outputs
Outputs for signal conditioning products can be voltage, current, frequency, timer or counter, relay, resistance or potentiometer, and other specialized outputs.User Interface
Signal converters have several user interfaces available that allow the user to make adjustments to the system.- A front panel is a local interface with integral controls, a keypad, and/or display on the panel of the unit
- Computer programmable converters are interfaced with a separate supervisory or host computer.
- Touch screens have a visual display which interacts with the user through touch. The user can directly put in information through the contact-sensitive screen.
- Remote and handheld devices can be mobile while the user enters program parameters.
_________________________________________________________________________________
ROBOTICS WITH SIGNAL CONDITIONER

Motor Controllers & Amplifiers
different types of motors and feedback options, now I want to talk about how to control those motors. Each motor is often refereed to an “axis”, so a 8 axis motor controller can control up to 8 motors. There are two different components used in the control of motors and people often use the terms interchangeably.

Amplifier (amp) or Drivers
This is the subject that actually makes the motor spin and contains (usually) the FET’s to allow motion to occur. This is the part that needs to rated for the power that you will use spinning your motors. There are many types of amplifiers you can purchase and each motor type (brushless, stepper, etc..) will often require a different type of amplifier. One notable exception is that many brushless motor amplifiers can often be configured to control a brushed DC motor. For brushless motors the hall sensors usually connect here to the amplifier.There are two types of amplifier inputs for issuing motor commands.
Analog – The drive takes an analog input signal to determine the speed of the motor. To change direction some amplifiers will have a separate direction bit that needs to be set or will want a negative analog voltage.
Digital – Digital amps will typically use higher level protocols and accept commands. The commands that can be issued digitally are typically more advanced than in an analog drive. Some common protocols for talking to a digital amplifier include serial, CAN, and etherCAT. Often a digital amplifier can also accept an analog signal.
Controller
This is the device that tells the amplifier what to do. Often encoders or other feedback devices will go into the controller which can then command the amplifiers for the desired position, velocity, or torque control. Other more advanced controllers can often be used that rely on various inputs, conditions, and pre-determined motions. Some examples of things that can be connected to a controller are secondary encoders, brakes, and limit switches.Hybrid
There are many devices that combine the amplifier and controller into a single integrated unit. We will be discussing these in more detail below. Many of the integrated controller and amplifiers also have an output to control an external amplifier.Within motor control systems there are two high level architectures. The first is a distributed system where each motor amplifier just controls a single motor and can be placed near that motor. The second type is a centralized system where all of the motor control decisions and amplifiers are in a single location. And as with everything else there is the hybrid option which is very common. In the hybrid option there is a separate amplifier/controller for each motor and then a separate higher level computer/controller that controls the coordination between the axis (remember axis=motor). Some of the deciding factors between the different architectures are:
Simplicity – The centralized option is often is simpler to program and coordinate motions between the various axis. In the centralized mode if the computer commands drive arcs or high level motions the centralized controller can determine the commands for each motor. Having a central controller lets each axis of motion know what the other axis are doing. It also can make integrating fail safes easier.
Wiring – This is often where distributed options win. In a centralized option if you are controlling a brushless motor with hall sensors and an encoder you can have 3 high power wires and 8 (5 for the encoder and 3 for the hall sensors) low power wires that need to be routed. In a distributed system you might only have 2 high power lines and 2 data lines (for example a CAN bus) that can be jumped between each amplifier (bus configuration instead of a star topology). If you have secondary encoders, limit switches, etc.. the wire count for the centralized option get worse while the distributed options remains the same. If you are trying to minimize wire count in a joint or running through the robot the distributed option is a clear winner. Also with the centralized option since all of the wires come to a single point it can be a large trunk of wires entering the controller that needs to be managed and possibly strain relieved.
Volume – In a centralized system you often have a single large block that needs to be mounted. In a distributed option the total volume might increase however it is in multiple blocks that can be moved around the robot and placed independently. This is not a pro or con for either approach, you need to determine which is more important is your system.
Heat Sinking – Motor amplifiers usually need to be heatsinked to a metal (thermally conductive) surface. Similar to the volume consideration above, where is it easier to get more thermal heatsinked surfaces in your robot.
Centralized Architecture Systems
Galil Motor Control
The Galil motor controller is my first choice when I want a single centralized controller. The 41×3 lets you connect up to 8 axis of motion to a single controller. To start you choose your controller board and how many axis you want. Once you have that there are different boards/amplifiers that can connect to the controller board allowing you to control different types of motors (up to 750W) or get more I/O. If you do not want to use their amplifiers you can use this controller with many of the analog amplifiers below. The controller comes with several analog and digital inputs and outputs based on which model you choose. You can also get a shunt regulator integrated into the controller which is an awesome feature. One of the really nice things I like about Galil is the prices are available online and they do not require you to call them for a quote.
The free Windows based user interface is pretty bare but I like it. It shows you the state of the different variables (speed, faults, etc..), gives you a code editor, and a terminal to type commands. The commands are pretty simple. For example to start a motor you might type “BG” to start motion on all axis or “BGA” to start motion on just axis A. The interface allows you to upload the code that is running on the controller which is a very valuable feature.
Writing a Linux driver for it was pretty easy as it is basic ASCII commands. They also provide code that can be used from Windows, Mac OSX, and Linux to get started with development but I have not used their code.
The biggest downside to the Galil is how it handles errors. I have seen many cases where an axis would have an error and using the saved error variables we could not determine what happened. Other annoying things is that comments in the code can affect timing and variables names in the code must be less than 8 characters. When the code fails you will often now know why it fails and you need to track it down on your own. Having a variable name longer then 8 characters has caused several of my programs to fail and require me to spend time debugging. Another thing the Galil lacks is a good method for determining if the wiring of a brushless motor is correct. Every company that makes motors and controllers has a different idea about which phase is which. Getting the correct combination on the Galil often takes a little trial and error.
The Galil learning center is also good and you should watch some of the videos.
Distributed Architecture Systems
Elmo Motor Controls
The Elmo Motor controllers is a large family of controllers with integrated amplifiers that can fit many different input voltages and operating power. They have controllers from 12V to 780V and controller that can handle 65KW. The drives can accept just about any type of interface and feedback type. There are three main lines of controls; simpleIQ, ExtrIQ (rugged version), and gold (faster, more feedback options, and supports etherCat). If you wish to use a Elmo centralized controller there is a Maestro controller you can use (I used an earlier model of the Maestro that had reliability and speed issues). Each controller also has several analog and digital IO (again based on the model you choose).
I am not sure how or why marketing types choose to name the drives things like Whistle and Drum and not something meaningful !$$%##%%!
The interface is pretty advanced and allows you to configure the drives and create programs to run in the drives. There is an ASCII based protocol for the Elmo drives that takes two letters and some options. (This can get confusing when you are working with both a Galil and an Elmo. For example to set the velocity one uses “JG” and the other uses “JV”.) When commanding multiple motors on a bus (for example with CANopen) you need to integrate CAN which can be a challenge.

I typically use the PCAN adapter to connect my computer to the CAN bus if there is no native interface. There are two modes (that I care about) for commanding motors via the CAN bus. The first allows you to send the ASCII commands in a CAN message to a RPDO (receive data object). The second method lets you set things directly using normal CANopen methods. This second option is more flexible and faster however it is harder to implement the software driver for this second approach.
Copley Controls

Click here for a full review of a Copley motor controller.
Copley is a newer player in the game however I have been impressed by their products. They are very similar to the Elmo’s however the cost is a little lower and the lead time is often shorter. The input voltage for the DC drives is from 20-180V and has a power rating of 2.7KW. Just like Elmo there are three classes of products; the standard drives, the plus versions, and the r-series rugged version. However within each class there are subclasses based on the type of motor you want to control. Thankfully the marking types did not create crazy names and stuck with meaningful names. For example a “ACM-055-18” is an accelnet (the family of the device) module (the form factor) with an input voltage range up to 55 volts and a peak current of 18 amps. As with the others there are several analog and digital IO on each drive.
The Copley interface can also be complicated like the Elmo one. You can program the controller and save routines in the controller (similar to the Galil and Elmo). Creating a driver for the Copley is really nice since you can communicate with one of the drivers over serial (RS-232) and then that driver can forward the CAN commands to all of the other drivers. This gives you the benefits of CAN with the simplicity of a serial protocol (I really like this feature!). Just like the other drives there is a basic ASCII protocol that can be used to talk to the drives.
Another nice thing is the Copley’s software process for setting up a new axis. While other controllers have this feature I like Copley’s the best. It has a process for auto detecting which phase of a brushless motor is which and can usually tell you how to fix the phases if needed (there is also a list of how to wire common motors. It also has an auto-tuning module that seems to work pretty good.
Their documentation for implementing CANopen is pretty bad.
Amplifiers (some with simple controllers)
Some of these amplifiers only take hall sensors and people will often try to commutate the motor based on that. However if you want reliable position and/or velocity control you will still want an encoder going to your controller.JR Kerr (digital)

The JR Kerr motor controllers takes a simple serial ASCII protocol and can send commands to different types of motors. The JR Kerr controllers are wired to each other in a bus which can simplify wiring and allow for the amps to be distributed in the robot. Unlike many of the other amps in this section this one can accept encoders to allow for position and velocity control. These operate at 12-48VDC and up to 6A continuous. The boards communicate via RS-485 and they sell as USB<->RS-485 adapter that fits with the amplifier stack up.
One downside to JR Kerr is that it is a small operation and has a higher risk of not being able to supply products then the large manufacturers.
Elmo (analog) – These drives take a +/-10VDC control signal where the positive or negative value determine the direction. The control is toque (current) based and is proportional to the input analog voltage. The only feedback these support is hall sensors for commutaing brushless motors.
Copley (analog) – The Copley analog amplifiers is pretty much the same as I wrote for the Copley analog amps however they have a smaller voltage and current rating.
Advanced Motion Controls (analog)

A-M-C is a great company for getting analog drives from. They have a wide selection and have many of them in stock for minimal lead time. They have a line of products called the MicroZ which are 1.5″ x 1.5″ (nice and small). I have often used a Galil controller card (with no amplifiers) to control these A-M-C amplifier cards. They have drives that can be controlled via the standard +/-10V commands, and a series of drives that use a PWM signal plus a direction line. Some of the drives can also accept an encoder for doing velocity commands as well as torque.
I have two complaint about the drives; the first is that there should be more input protection. I had a case where a fuse blew allowing the line voltage to increase which killed one of the commutating phases (probably a blown FET) (to their credit it was right before a holiday when most people went home and they stayed on the phone to help me and process an RMA, so +10 for customer service). The second is that there are a couple of potentiometers used to configure the amp that could be labeled better.
_________________________________________________________________________________
Gen. Mac Tech Zone MARIA PREFER with TRANS___OP
__________________________________________________________________________________
Tidak ada komentar:
Posting Komentar